S.M. Abidur Rahman, Billal Hossain Momen, Rashedur Rahman Tanvir, Bitopi Biswas, Mosammat Nilufar Yasmin, Tariful Alam Khan, M. Robiul Islam
ABSTRACT. A hydroponic experiment was conducted at the Precision and Automated Agriculture Laboratory, Department of Agronomy and Agricultural Extension, University of Rajshahi, from April to August 2023. The objective was to investigate the morphological and physiological responses of maize to varying nitrogen sources and stress levels in hydroponic systems. The experiment comprised three nitrogen treatments: CN (100% chemical nitrogen as 2 mM NH4NO3), ON (100% organic nitrogen as 4 mM glycine), and LN (low nitrogen as 10% of 2 mM NH4NO3 chemical nitrogen solution). The popular maize variety NH7720 (marketed by Syngenta Bangladesh Limited) was used. The experiments followed a completely randomised design with three replications. The CN treatment consistently outperformed the ON and LN treatments in various growth-related parameters, including plant height (72.73 cm), leaf area (295.54 cm²), shoot dry weight (0.65 g/plant), total chlorophyll content (3.11 mg/g), and shoot (11.06%) and root (10.82%) protein content, indicating that adequate nitrogen treatment stimulated strong growth and development in maize plants. Conversely, the LN treatment exhibited a superior shoot-to-root ratio (85.43%), proline accumulation (188.01 µg/g), number of root tips (21.25), root length (31.65 cm), root network area (619.10 cm²), root diameter (5.63 mm), root volume (13944.71 mm³), and root surface area (3705.51 mm²). These results suggest that under nitrogen-deficient conditions, maize plants allocate resources to root development and stress tolerance mechanisms. The organic nitrogen (ON) treatment showed intermediate results, being statistically similar to both the CN and LN treatments across a range of characteristics, suggesting that organic nitrogen or glycine might be less effective than chemical nitrogen or ammonium nitrate in promoting optimal maize growth.
Keywords: hydroponic experiment; maize; morphological adaptation; nitrogen stress; physiological adaptation.
Cite
ALSE and ACS Style
Rahman, S.M.A.; Momen, B.H.; Tanvir, R.R.; Biswas, B.; Yasmin, N.M.; Khan, T.A.; Islam, M.R. Morphological and physiological responses of maize to varying nitrogen sources and stress levels in hydroponic systems: a comparative study. Journal of Applied Life Sciences and Environment 2024, 57, 385-401.
https://doi.org/10.46909/alse-573143
AMA Style
Rahman SMA, Momen BH, Tanvir RR, Biswas B, Yasmin NM, Khan TA, Islam MR. Morphological and physiological responses of maize to varying nitrogen sources and stress levels in hydroponic systems: a comparative study. Journal of Applied Life Sciences and Environment. 2024; 57 (3): 385-401.
https://doi.org/10.46909/alse-573143
Chicago/Turabian Style
Rahman, İ.S.M., Abidur, Billal Hossain Momen, Rashedur Rahman Tanvir, Bitopi Biswas, Mosammat Nilufar Yasmin, Tariful Alam Khan, and M. Robiul Islam. 2024. “Morphological and physiological responses of maize to varying nitrogen sources and stress levels in hydroponic systems: a comparative study” Journal of Applied Life Sciences and Environment 57, no. 3: 385-401.
https://doi.org/10.46909/alse-573143
View full article (HTML)
Morphological and Physiological Responses of Maize to Varying Nitrogen Sources and Stress Levels in Hydroponic Systems: a Comparative Study
S.M. Abidur RAHMAN, Billal Hossain MOMEN, Rashedur Rahman TANVIR, Bitopi BISWAS, Mosammat Nilufar YASMIN, Tariful Alam KHAN and M. Robiul ISLAM*
Farming Systems Engineering Laboratory, Department of Agronomy and Agricultural Extension, Rajshahi University, Rajshahi, Bangladesh; email: shohagru18@gmail.com; mdbillalhossainmomen83@gmail.com; rashedtanvir100@gmail.com; bitopi38ru@gmail.com; nyasmin@ru.ac.bd; tariful_khan@ru.ac.bd
*Correspondence: mrislam@ru.ac.bd
Received: May 21, 2024. Revised: Jul. 03, 2024. Accepted: Jul. 19, 2024. Published online: Aug. 01, 2024
ABSTRACT. A hydroponic experiment was conducted at the Precision and Automated Agriculture Laboratory, Department of Agronomy and Agricultural Extension, University of Rajshahi, from April to August 2023. The objective was to investigate the morphological and physiological responses of maize to varying nitrogen sources and stress levels in hydroponic systems. The experiment comprised three nitrogen treatments: CN (100% chemical nitrogen as 2 mM NH4NO3), ON (100% organic nitrogen as 4 mM glycine), and LN (low nitrogen as 10% of 2 mM NH4NO3 chemical nitrogen solution). The popular maize variety NH7720 (marketed by Syngenta Bangladesh Limited) was used. The experiments followed a completely randomised design with three replications. The CN treatment consistently outperformed the ON and LN treatments in various growth-related parameters, including plant height (72.73 cm), leaf area (295.54 cm²), shoot dry weight (0.65 g/plant), total chlorophyll content (3.11 mg/g), and shoot (11.06%) and root (10.82%) protein content, indicating that adequate nitrogen treatment stimulated strong growth and development in maize plants. Conversely, the LN treatment exhibited a superior shoot-to-root ratio (85.43%), proline accumulation (188.01 µg/g), number of root tips (21.25), root length (31.65 cm), root network area (619.10 cm²), root diameter (5.63 mm), root volume (13944.71 mm³), and root surface area (3705.51 mm²). These results suggest that under nitrogen-deficient conditions, maize plants allocate resources to root development and stress tolerance mechanisms. The organic nitrogen (ON) treatment showed intermediate results, being statistically similar to both the CN and LN treatments across a range of characteristics, suggesting that organic nitrogen or glycine might be less effective than chemical nitrogen or ammonium nitrate in promoting optimal maize growth.
Keywords: hydroponic experiment; maize; morphological adaptation; nitrogen stress; physiological adaptation.
INTRODUCTION
Maize (Zea mays L.) is one of the most frequently cultivated crops in the world, often called the “Queen of grains” due to its short growing season, high yield potential, and nutrient content (Begam et al., 2018). People may utilise it as a source of carbohydrates and protein and as a source of animal feed and raw materials for various industrial goods and cooking ingredients (Ayyar et al., 2019). Furthermore, maize consumption has been related to a number of health benefits, including lower blood cholesterol, improved kidney and bone function, and protection against cardiovascular disease, diabetes, and hypertension (Islam and Hoshain, 2022).
Nitrogen is necessary for plant growth, and its availability significantly limits agricultural production (Fathi and Zeidali, 2021). Nitrogen is essential to plant proteins, nucleic acids, nucleoproteins, chlorophyll, enzymes, alkaloids, and plant hormones (Wen et al., 2020). Most nitrogen taken by plants is NO3−, which is also the nutritional element that absorbs the fastest. Nitrate is the primary form of nitrogen for most plant life, making it essential for growth, development, and morphological maturation (Wang et al., 2020). To produce 1 kg biomass, most of the non-legume plants absorb 20–50 g of N from the soil (Ohyama et al., 2017). Approximately 1011 kg N per acre per annum is applied in the soil globally with the aim of achieving the highest crop production (Govindasamy et al., 2023). Therefore, it has been demonstrated that with higher chemical nitrogen application, the crop yield will increase (Anas et al., 2020). However, high levels of soil nitrogen result in losses, ultimately reducing the nitrogen use efficiency (NUE) (Whetton et al., 2022). To increase the NUE, organic nitrogen is used instead of chemical nitrogen. Organic nitrogen releases nitrogen slowly in the soil; for that reason, plants can absorb nitrogen for a long period of time. To identify the physiological responses of maize plants, different concentrations of chemical nitrogen and organic nitrogen were used for this experiment.
Nitrogen stress in plants can result from an excess or shortage (Kong et al., 2017). In the vegetative and reproductive stages, phenological development is delayed by a nitrogen deficit. The nitrogen supply and photosynthesis are closely related. A nitrogen deficit reduces photosynthesis and the green leaf area, decreasing plant productivity (Fan et al., 2022). Most plants experience a decline in photosynthetic rates under severe nitrogen stress conditions. First, for a reduction in mesophyll cells and bundle sheath cells, stomatal conductance affects the intercellular CO2 concentration. The second factor is the lower level of bioenergetics and light-harvesting proteins, which slow the rate of electron transfer and increase the amount of light energy converted to heat. The third factor is the reduced activity of photosynthetic enzymes, which slows the carboxylation rate (Mu and Chen, 2021). In reaction to insufficient nitrogen transportation from the environment, plants predominantly modify their root systems (Briat et al., 2020). Most plants extend their roots to absorb nitrogen through rhizosphere exploration. As a highly mobile element, nitrogen is quickly lost after it has been applied to the soil in an inorganic form. In contrast, nitrogen production in organic forms is slower and needs to catch up with plant demands (Khan et al., 2019).
Maize exhibits both morphological and physiological adaptations to cope with nitrogen stress conditions (Hasibuzzaman et al., 2021). Maize plants can alter their root architecture to explore a larger soil volume for nitrogen absorption (Schneider et al., 2021). When nitrogen is limited, maize plants tend to develop a more extensive root system, including lateral roots and root hairs, to increase their access to available nitrogen sources. In response to nitrogen stress, maize plants often reduce the size and number of leaves (Hemati et al., 2022). This leaf area reduction helps to conserve nitrogen for essential functions, such as chlorophyll and protein synthesis. Under nitrogen stress, maize plants may undergo premature leaf senescence, shedding older leaves to salvage nutrients and directing them towards developing grains and essential tissues (Bhattacharya et al., 2022).
Maize plants make physiological adjustments to enhance nitrogen uptake and transport (Hui et al., 2022). This entails optimising nitrogen allocation to reproductive structures, such as kernels, which receive priority, while non-essential vegetative growth receives less nitrogen (Chen et al., 2023). Maize can adjust its nitrogen assimilation processes by regulating the expression of nitrogen metabolism-related genes (Peng et al., 2023). When nitrogen is scarce, maize plants can increase their nitrogen assimilation efficiency to make the most of the available nitrogen (Gu, 2023). Drought stress can often occur in conjunction with nitrogen stress. Maize has developed mechanisms for drought tolerance, such as reduced transpiration rates and increased water-use efficiency, which can indirectly help plants deal with nitrogen stress (Chieb and Gachomo, 2023).
Plants typically absorb nitrogen in the form of NO3− and NH4+, although they can also use organic nitrogen molecules. Inorganic nitrogen sources such as nitrate, ammonium, and urea are easily accessible and encourage rapid development. Compost, manure, blood meal, and bone meal are organic sources of nitrogen that provide a slow-release form, while also improving soil structure and microbial activity (Shaji et al., 2021). Specialised nitrogen sources, such as controlled-release fertilisers and nitrogen-fixing crops, provide extra benefits by releasing nutrients gradually and increasing soil fertility. Diverse nitrogen sources improve soil health and sustainability, increase plant growth and production, improve nutrient absorption, and reduce environmental pollution by reducing runoff and leaching (Saha et al., 2020). Farmers and hydroponic growers may obtain healthier plants, increased yields, and a more sustainable and ecologically friendly approach to agriculture by incorporating a variety of nitrogen sources. By reusing waste and lowering reliance on synthetic fertilisers, which have the potential to harm the environment, organic nitrogen sources promote sustainable farming (Rodríguez-Espinosa et al., 2023). In addition, they activate good soil bacteria that support nutrient cycling and prevent illness. Organic nitrogen is a sustainable and environmentally beneficial option for gardening and agriculture since it enhances plant health, lowers the need for chemicals, and increases soil fertility in the long term (Baweja et al., 2020).
The specific adaptations of maize under nitrogen stress may vary depending on the genetic characteristics of the maize variety, the nitrogen stress severity, and other environmental factors (Dao et al., 2023). Studies on the morphological and physiological adjustments of maize to nitrogen stress can provide valuable insights into the complex interactions between plants and nutrients. This knowledge can help guide the development of long-term agricultural practices that promote nutrient stewardship while minimising environmental impacts (Liu et al., 2023).
The present study was therefore undertaken to examine alterations in root architecture, shoot growth, leaf morphology, and the overall structure of the plant under variable nitrogen availability.
MATERIALS AND METHODS
Plant materials and growth conditions
This study was conducted in the hydroponic culture chamber of the Precision and Automated Laboratory, Department of Agronomy and Agricultural Extension, the University of Rajshahi, in 2023 (Figure 1).
The standard growth chamber had a controlled alternate day/night temperature of 28/22°C, humidity level above 65%, and light intensity of about 300 µmol photons m−2 s−1 illumination with a 14/10-h (day/night) photoperiod and proper aeration. The experiment consisted of three nitrogen treatments: CN (100% of 2 mM NH4NO3 chemical nitrogen), ON (100% organic nitrogen), and LN (10% of 2 mM NH4NO3 chemical nitrogen as low nitrogen). The popular hybrid maize variety NH7720 (marketed by Syngenta Bangladesh Limited, Dhaka) was used for the experiment. The experiments were conducted in a completely randomised design with three replications. Seedlings of uniform size were transplanted into sterile foam-plugged apertures in a plastic lid, which floated atop a nutrient solution within a plastic container.
Seedlings were transplanted when they reached the two-leaf stage. The comprehensive nutrient solution comprised a blend of substances aimed at fostering heightened biomass accumulation and eliciting hormonal modifications. This solution, referred to as (CN), contained the following: 2 mM NH4NO3, 0.75 mM K2SO4, 0.25 mM KH2PO4, 0.1 mM KCl, 0.2 mM Fe-EDTA, 0.65 mM MgSO4, 2 mM CaCl2, 1×10−3 mM ZnSO4, 1×10−3 mM MnSO4, 5 × 10−6 mM (NH4)6Mo7O24, 1×10−4 mM CuSO4, and 1×10−3 mM H3BO3. NH4NO3 was substituted with a higher concentration of 4 mM glycine (C2H5NO2) to serve as the source of organic nitrogen (ON).
The low nitrogen (LN) treatment was formulated with a solution comprising a 10% nitrogen source (2 mM NH4NO3), identical to that employed in the control (CN) treatment. The pH of the nutrient solution was adjusted to fall within the range of 5.8–6, with replacement of the nutrient solution occurring every 2 days. Root and shoot samples were harvested 21 days after transfer to the nutrient solution, and morphological traits were documented. For physiological assessments, half of the samples were promptly frozen in liquid nitrogen and subsequently preserved at −80°C. The remaining half of the samples underwent oven drying to determine the dry weight (DW) and to conduct additional biochemical analyses, such as protein content determination.
Morphological parameters
After an extended period of 21 days following transplant, the height of the three plants, picked at random, was measured from the base of the plant to the tip of the tallest leaf. The height of the plants was measured in centimetres, and the average value for each pot was calculated.
The leaf area was determined by measuring the length and width of the three leaves from each plant. Shoot and root dry weights were determined after they had been completely dried in an oven.
To determine the root architecture, the root system was partitioned into the crown and primary roots. Each root type was manually quantified, and the length of the primary roots was measured using a ruler. Subsequently, the roots were scanned using the Epson Expression 1680 Flatbed scanner.
Analysis of the scanned images was performed utilising RhizoVision Explorer software (version 2.0.3) to obtain metrics, such as the number of root tips, network area, average diameter, root volume, and surface area (Figure 2).
Physiological parameters
The leaf chlorophyll content was quantified utilising 100 mg of fresh weight (FW) from fully expanded frozen leaves. Subsequently, the sample underwent homogenisation with acetone, followed by incubation in the dark for 24 hours.
After this incubation period, the sample was subjected to centrifugation at 10,000 rpm for 10 minutes at 4°C, and absorbance readings were recorded at wavelengths of 645 and 663 nm. The chlorophyll content was then determined using a specified formula and expressed as milligrams per gram of fresh weight (mg g−1 FW) of the leaf.
The proline concentration was determined according to the methodology outlined by Bates et al. (1973). Frozen leaf tissue underwent homogenisation in 3% sulphosalicylic acid, followed by centrifugation at 11,500×g for 15 minutes. The resulting supernatant was then combined with acid ninhydrin and glacial acetic acid and heated in a water bath at 100°C for 60 minutes. The reaction was terminated by placing the tube in an ice bath for 15 minutes. Following cooling, 2 mL of toluene was introduced to the reaction mixture and vortexed for 20–30 seconds. The optical density of the resulting chromophore-containing toluene was measured spectrophotometrically at 520 nm, with toluene serving as a blank. The proline concentration was subsequently determined from the standard curve utilising laboratory-grade proline.
To ascertain the protein content in both shoot and root tissues, the dried samples underwent grinding and sieving through a 1-mm mesh prior to analysis. The nitrogen content was quantified utilising the Kjeldahl method (AOAC, 1990), and the crude protein content was subsequently calculated by multiplying the nitrogen value by 6.25.
Statistical analysis
Analysis of variance was conducted using STATVIEW software. Treatment mean differences were assessed using Duncan’s Multiple Range Test (DMRT) at significance levels of 5 and 1%.
RESULTS
Plant height
The plant heights of the maize variety were assessed at 21 days after transplant (DAT) to the hydroponic system. Discernible disparities in plant height were observed under different nitrogen treatments (Table 1). Specifically, the plants subjected to the CN treatment demonstrated the tallest average height, reaching 72.73 cm. In contrast, those subjected to the LN treatment exhibited the shortest height, measuring 54.61 cm. The plant height under the LN treatment was 24.91% lower than that under the CN treatment.
Leaf area
The leaf area of maize (measured in cm2) showed significant variations across different nitrogen doses, as shown in Table 1.
The CN treatment exhibited the largest leaf area, measuring 295.54 cm2. This measurement was statistically comparable to the leaf area under the ON treatment, but it was notably 25.07% higher than the leaf area observed under the LN treatment. Conversely, the leaf area observed under the LN treatment was the smallest, measuring 221.46 cm2.
Shoot dry weight
There was a significant discrepancy in the dry mass of maize shoots (g/plant). The maximum shoot dry weight was recorded at CN (0.65 g/plant), demonstrating statistical equivalence with ON (0.57 g/plant). Conversely, LN displayed a reduced value (0.47 g/plant), marking a notable 27.69% decline compared to CN (Table 1).
Root dry weight
The root dry weight (g/plant) of maize exhibited significant variation under nitrogen stress conditions, as shown in Table 1. The highest root DW was recorded under LN conditions, with a mean value of 0.40 g per plant. Although this value was not significantly different from the root DW observed under ON conditions, which was measured at 0.33 g per plant, there was a notable discrepancy when compared to the root DW under CN conditions. Under CN conditions, the root dry weight was lower, measuring 0.28 g per plant.
Shoot–root ratio
This study examined the fluctuations in the shoot–root ratio (%) among the three nitrogen treatments. The lowest shoot–root ratio was recorded in the LN treatment, at 85.43%. Conversely, the shoot–root ratio was notably lower in the CN treatment at 42.55%, displaying significant variance when compared to the ON treatment at 58.49% and the LN treatment (Table 1).
Root architecture
Root length
A comprehensive portrayal of the notable discrepancies in root length (measured in centimetres) resulting from the application of different nitrogen levels is presented in Table 2. Under LN conditions, roots exhibited remarkable growth, achieving an impressive length of 31.65 cm. However, this length experienced a slight reduction of 14.15% under ON conditions, indicating a modest decline in overall root development. Moreover, under CN treatment, the root length displayed a substantial decrease of 34.03%, signifying a notable decline in root growth under these conditions.
Number of root tips
The observed variation in the number of root tips was statistically significant compared to the three distinct nitrogen doses, as shown in Table 2. LN treatment had the highest count of root tips (21.25), whereas the CN treatment had the lowest count (10.65). Consequently, the LN treatment resulted in a significant decrease of 21.6 and 49.88% compared to the ON and CN treatments, respectively.
Root network area
The root network area of maize plants, quantified in square centimetres (cm2), displayed notable fluctuations due to varying nitrogen frequencies, as shown in Table 2. The smallest observed network area, which was observed in CN, exhibited an impressive growth increase of 26.09% in ON and 42.38% in LN.
Root diameter
The utilisation of various nitrogen dosages resulted in discernible effects on the average root diameter (mm) in maize. The LN treatment demonstrated the most remarkable average diameter, registering 5.63 mm. ON treatment resulted in an average diameter of 4.45 mm. CN treatment resulted in the smallest average diameter, measuring 3.67 mm, as shown in Table 2. This observation indicates a significant reduction of 34.81% in the LN group compared to the CN group.
Root volume
In relation to the maize root volume (mm3), the impacts of nitrogen treatment exhibited significant variations, as shown in Table 2. The highest volume observed (13,944.71 mm3) was found in LN, while ON had a lower volume and CN had a significantly lower volume, with reductions of 17.76 and 52.28%, respectively.
Root surface area
The nitrogen treatments resulted in significant variations in the root surface area (mm2) in maize. As shown in Table 2, LN exhibited the highest surface area, measuring 3705.51 mm2. In contrast, CN displayed the lowest surface area, measuring 1670.81 mm2. In LN, there was a significant reduction of 54.91% in the highest root surface area observed compared to CN.
Chlorophyll content
Figure 2 illustrates the chlorophyll contents (chlorophyll a, chlorophyll b, and total) in maize leaves.
Table 1
Growth parameters of maize under different nitrogen treatments
Treatment |
Plant Height (cm) |
Leaf Area (cm2) |
Shoot DW (g/plant) |
Root DW (g/plant) |
S/R % |
CN |
72.73±2.34a |
295.54±9.65a |
0.65±0.04a |
0.28±0.01b |
42.55±1.67c |
ON |
63.88±2.75ab |
248.36±13.46ab |
0.57±0.05ab |
0.33±0.02ab |
58.49±1.30b |
LN |
54.61±3.01b |
221.46±13.12b |
0.47±0.04b |
0.40±0.02a |
85.43±3.21a |
LS |
0.01 |
0.01 |
0.01 |
0.01 |
0.01 |
In each column, different lowercase letters show significant differences between treatments based on DMRT. Values with the same letter or without letters do not differ significantly. Values show the mean ± standard error (SE) of three replicates. LS = level of significance, CN = 100% chemical nitrogen (recommended dose), ON = 100% organic nitrogen, LN = 10% chemical nitrogen
Table 2
Root length and root architecture of maize under different nitrogen treatments
Treatment |
Root Length (cm) |
Number of Root Tips |
Network Area (cm2) |
Average Root Diameter (mm) |
Root Volume (mm3) |
Root Surface Area (mm2) |
CN |
20.88± 1.24b |
10.65± 0.80c |
356.73± 13.83c |
3.67± 0.21b |
6654.41± 581.10b |
1670.81± 279.16b |
ON |
27.17± 1.23a |
16.66± 0.82b |
482.68± 19.21b |
4.45± 0.25b |
11468.55± 674.79a |
2811.81± 360.07ab |
LN |
31.65± 1.46a |
21.25± 0.70a |
619.10± 23.99a |
5.63± 0.23a |
13944.71± 779.63a |
3705.51± 317.77a |
LS |
0.01 |
0.01 |
0.01 |
0.01 |
0.01 |
0.01 |
In each column, lowercase letters show significant differences between treatments at P<0.05 based on DMRT. Values with the same letter or without letters do not differ significantly. Values show the mean ± standard error (SE) of three replicates. LS = level of significance, CN = 100% chemical nitrogen (recommended dose), ON = 100% organic nitrogen, LN = 10% chemical nitrogen.
The highest observed chlorophyll a content occurred under CN treatment (2.18 mg/g). Conversely, the lowest chlorophyll a content measurement (1.67 mg/g) was observed in LN. For chlorophyll b, CN treatment exhibited the highest concentration at 0.93 mg/g. However, this value showed a slight decrease of 17.20% in the ON treatment and a significant reduction of 24.73% in the LN treatment. The chlorophyll b content in LN reached its lowest level at 0.70 mg/g. CN had the highest total chlorophyll content at 3.11 mg/g. In contrast, the total chlorophyll content in LN was exceptionally low, measuring 2.38 mg/g. Although this value was statistically similar to ON, it differed significantly from CN.
Proline content
A notable divergence was observed in the proline content (µg/g) of maize leaves under different nitrogen treatments (Figure 3). The highest observed proline content (188.01 µg/g) was found in LN, which experienced a slight decrease of 18.93% compared to ON. However, there was a significant difference of 37.02% compared to CN. In the CN sample, the observed proline content was lowest (118.41 µg/g).
Protein content in shoots and roots
The shoot and root protein content (%) in maize differed significantly across the nitrogen treatments (Figure 4). The shoot protein concentration peaked (11.06%) under CN treatment, decreased by 11.93% in ON, and significantly decreased by 19.53% in LN. The root protein content was lowest in the LN treatment, at 8.58%. This value exhibited a statistically significant difference from both the ON and CN treatments, which recorded root protein contents of 10.05 and 10.82%, respectively. The CN treatment demonstrated a remarkable increase of 20.70% in root protein content compared to the LN treatment.
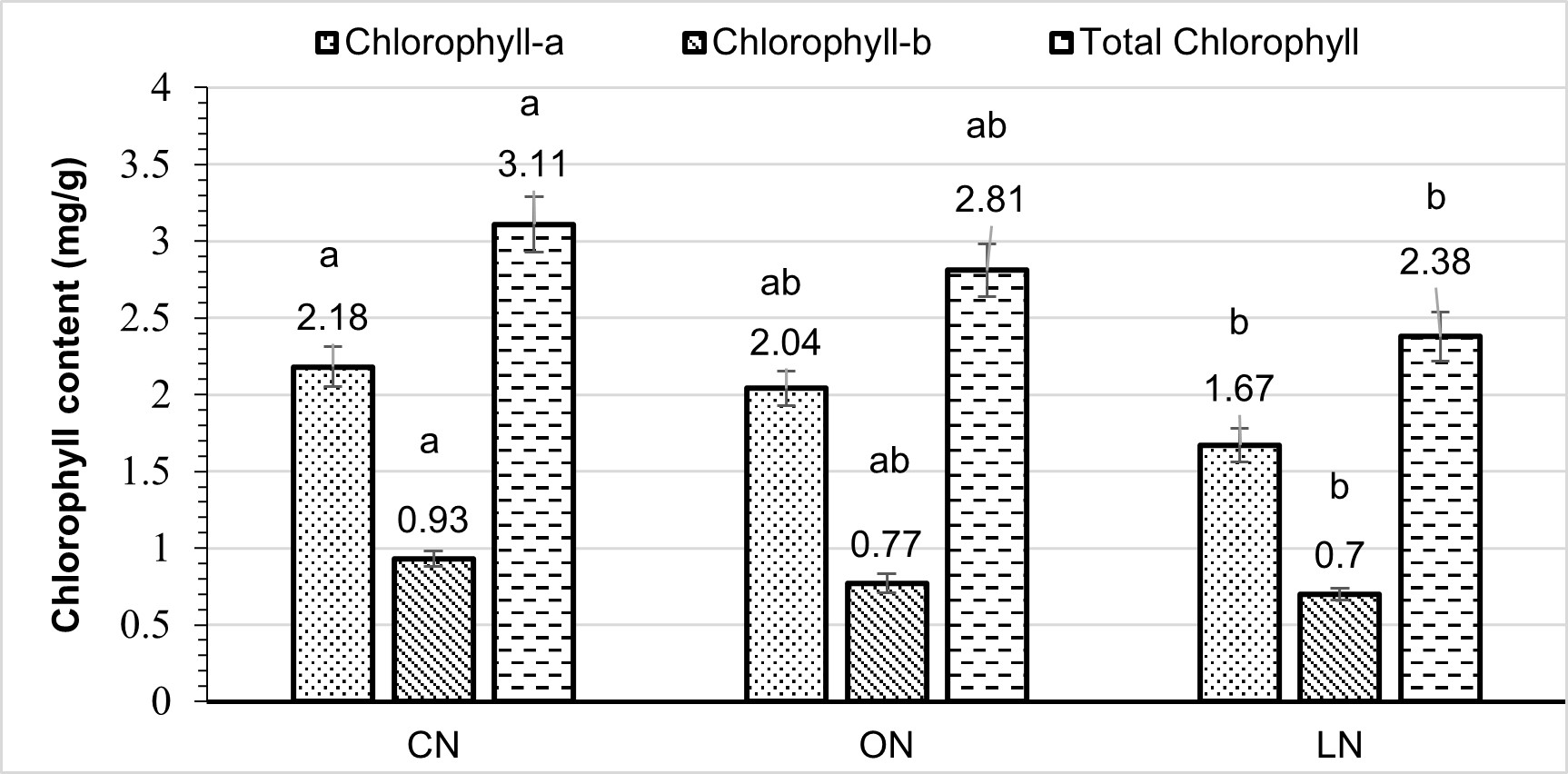
Figure 3 – Leaf chlorophyll content (mg/g fresh weight) under different nitrogen treatments
CN = chemical nitrogen, ON = organic nitrogen and LN = low nitrogen Small bars show standard errors; each bar marked with a different letter for chlorophyll content (a, b, and total chlorophyll) differed significantly
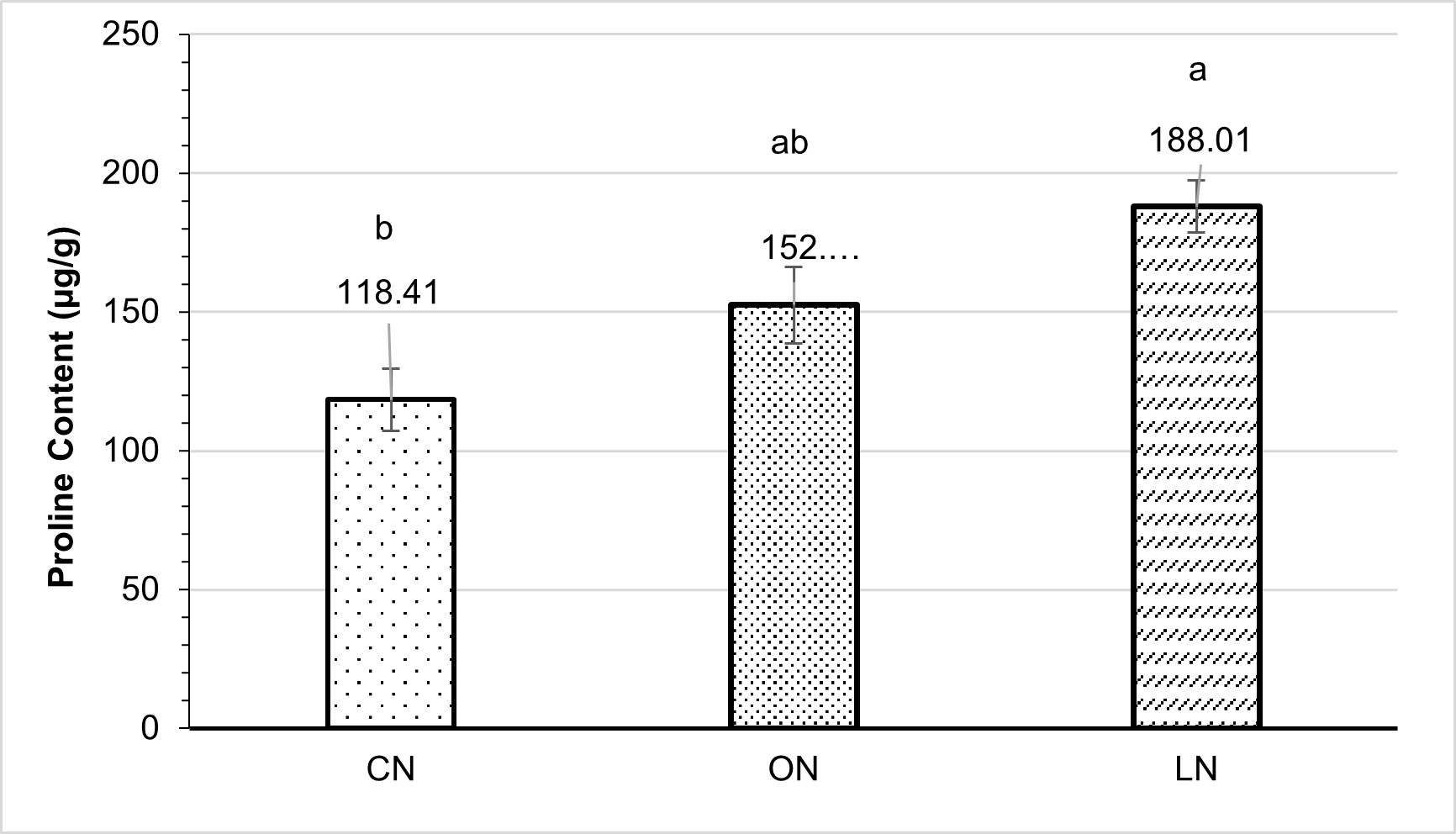
Figure 4 – Proline content (µg/g) of maize under different nitrogen treatments
CN = chemical nitrogen, ON = organic nitrogen, and LN = low nitrogen Small bars show standard errors; each bar marked with a different letter differed significantly
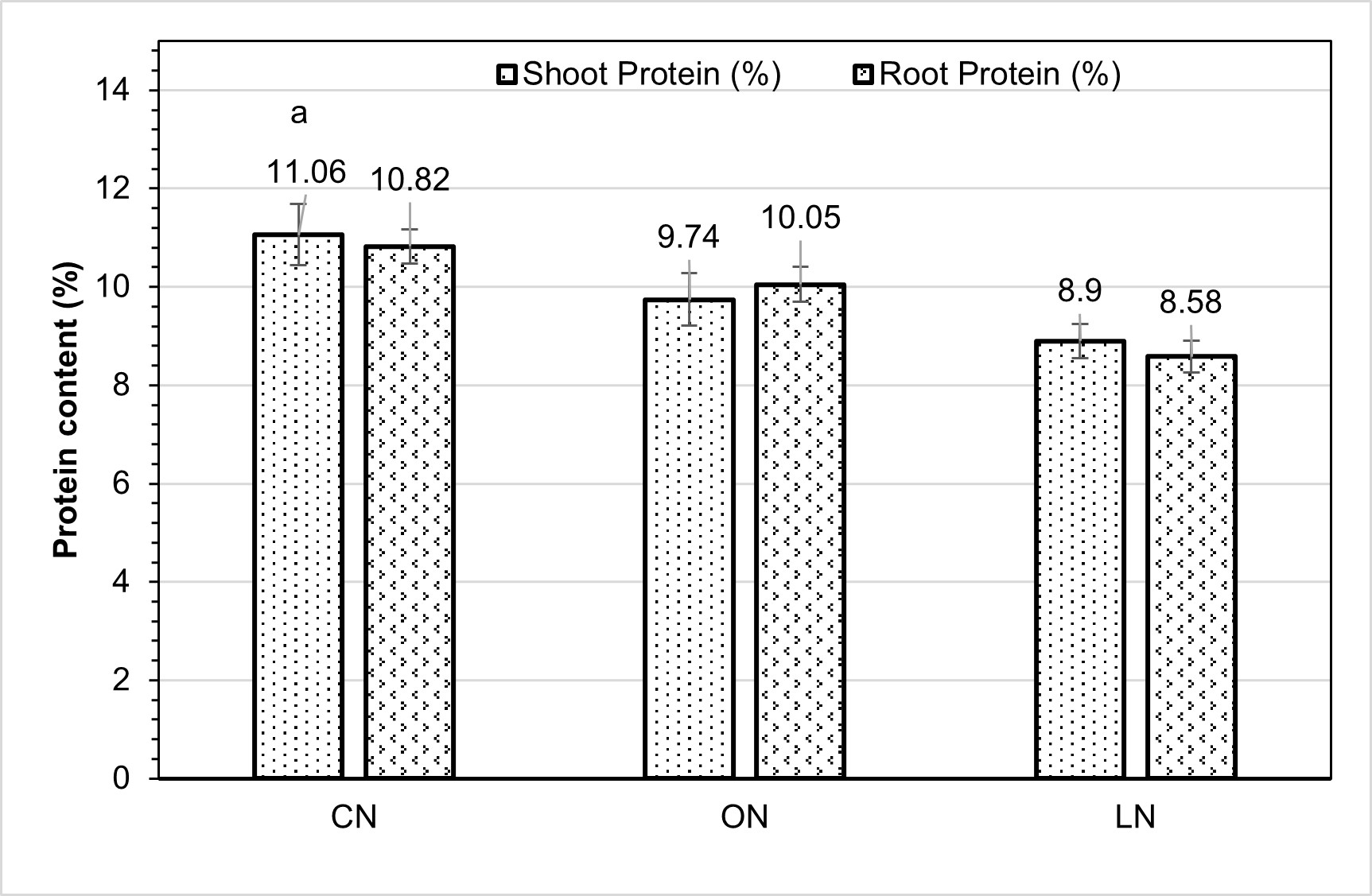
Figure 5 – Protein content (%) in maize shoots and roots under different nitrogen treatments
CN = chemical nitrogen, ON = organic nitrogen, and LN = low nitrogen. Small bars show standard errors; each bar marked with a different letter for the roots or shoots differed significantly
DISCUSSION
The results of the study provide useful insights into maize plants’ unique reactions to different nitrogen treatments. These findings provide insight into the complex relationship between nitrogen availability and several morphological and physiological characteristics in maize.
First, this study demonstrated that nitrogen availability has a considerable impact on plant height, a critical indicator of growth and development. Maize plants treated with chemical nitrogen (CN) had the highest average height, whereas those treated with low nitrogen (LN) had the shortest height (Table 1). This significant difference emphasises the role of nitrogen in fostering vertical growth and total plant stature. Insufficient nitrogen levels can cause stunted growth, as nitrogen is crucial for chlorophyll production, while elevated nitrogen levels can lead to increased height but a slender appearance. Nitrogen positively influences cellular proliferation and enlargement, promoting stem elongation. However, excess nitrogen can hinder maturation and grain yield (Sahoo et al., 2021; Zhao et al., 2022).
Similarly, the leaf area of maize plants differed between nitrogen treatments, with CN treatment resulting in the highest leaf area. This highlights the critical function of nitrogen in leaf expansion and canopy formation, which are required for efficient photosynthesis and biomass buildup. Insufficient nitrogen leads to a reduced leaf size and decreased surface area. Nitrogen is crucial for protein synthesis (Figure 5), facilitating cell growth and division. Elevated nitrogen levels allow maize plants to develop larger leaves and stimulate cell division, while an overabundance can lead to slender, elongated foliage (Ochieng et al., 2021; Zhang et al., 2020).
Furthermore, this study investigates the effects of nitrogen stress on the shoot and root biomass. Maize plants treated with CN had the highest shoot dry weight, indicating robust aboveground biomass production under ideal nitrogen circumstances. Conversely, the LN treatment resulted in a significant reduction in shoot dry weight, highlighting the negative impact of nitrogen limitation on aboveground biomass formation. The growth rate and dry weight of maize are influenced by the availability of nitrogen to the plant. In situations in which nitrogen levels are insufficient, maize plants tend to exhibit a decrease in their shoot and root dry weight (Su et al., 2020; Wang et al., 2019).
Interestingly, the root system reacted differentially to nitrogen stress, with LN treatment producing the highest root dry weight. This finding suggests a compensatory mechanism in which maize plants devote more resources to root growth in nitrogen-deficient environments, thereby improving nutrient uptake and resource acquisition (Wang et al., 2019).
The study also emphasises the importance of the root structure in nitrogen adaption. Maize plants treated with LN showed significant increases in root length, volume, and surface area compared to CN treatment (Table 2). The root structure tends to show the least response in organic nitrogen and high nitrogen compared to low nitrogen treatment. Organic nitrogen results in a moderate response in all root-related parameters (Khan et al., 2019). These adaptive responses demonstrate the plant’s ability to explore a broader soil volume and improve nutrient uptake efficiency in nitrogen-limited situations. Root length is crucial for a plant’s ability to absorb nutrients and water. Maize plants adapt to nitrogen scarcity by developing elongated roots and navigating deeper soil layers (Pan et al., 2023). However, an abundant nitrogen content in the topmost soil layer reduces root lengths, reducing the plant’s need for extensive root system growth. Chen et al. (2023) also observed similar findings.
Moreover, this study investigates the effects of nitrogen availability on chlorophyll and proline levels in maize leaves. The chlorophyll content, which is essential for photosynthesis and general plant vigour, varies across nitrogen treatments, with the CN treatment producing the greatest chlorophyll levels. There was a correlation between low nitrogen levels and decreased chlorophyll content in maize leaves (Figure 3) due to the presence of nitrogen in chlorophyll, as insufficient nitrogen levels hinder chlorophyll synthesis. Consequently, maize leaves deficient in nitrogen may appear yellow or chlorotic (Croft et al., 2020). Proline content, an indication of stress tolerance, increased after LN treatment, indicating a preventive mechanism against nitrogen stress-induced damage. The consequences of nitrogen deficiency, such as restricted growth and diminished yield, can be mitigated to some extent through proline accumulation (Figure 4). In contrast, when exposed to higher nitrogen levels, maize plants exhibit a decreased inclination to accumulate proline in their leaves (Mosaad et al., 2020).
Finally, this study emphasises the role of nitrogen in controlling protein synthesis in maize shoots and roots. Maize plants treated with CN had a higher protein content in both shoots and roots than plants treated with LN, demonstrating that nitrogen plays an important role in protein accumulation and metabolic activities required for growth and development. Nitrogen is a crucial element in protein formation. When there is a nitrogen scarcity, the plant gives priority to protein production in reproductive organs rather than in shoots, resulting in a reduction in the protein content of the shoots (Figure 5). In addition, the plant can redistribute resources from the process of creating proteins in the aboveground parts of the plant towards root growth, which helps the plant absorb more nitrogen. This, in turn, leads to a further decrease in protein levels in the aboveground parts of the plant (Song et al., 2019). Stress can make roots grow faster, which can cause a dilution effect in which the total protein stays the same but is spread out over a higher volume, lowering the protein quantity per unit weight. When plants are stressed, they may put more emphasis on proteins that help them take in nitrogen, which can cause these levels to rise (Yang et al., 2019).
CONCLUSIONS AND RECOMMENDATION
The experiment with three nitrogen treatments, CN (100% chemical nitrogen), ON (100% organic nitrogen), and LN (10% chemical nitrogen as low nitrogen), on the popular maize variety NH7720 yielded interesting results regarding the impact of nitrogen on several growth metrics. Based on the results, the CN treatment exhibited superior characteristics in plant height (72.73 cm), leaf area (295.54 cm2), shoot dry weight (0.65 g per plant), total chlorophyll content (3.11 mg per g leaf fresh weight), and shoot (11.06%) and root (10.82%) protein. However, the LN treatment resulted in peak conditions for shoot and root ratio (85.43%), proline content (188.01 µg/g), number of root tips (21.25), root length (31.65 cm), root network area (619.10 cm2), root average diameter (5.63 mm), root volume (13,944.71 mm3), and root surface area (3705.51 mm2).
The results showed that the CN treatment consistently outperformed the ON and LN treatments in terms of growth-related parameters, indicating that the regulated nitrogen treatment stimulated strong growth and development in maize plants. However, the LN treatment exhibited significant characteristics, including superior shoot and root ratio, proline content, number of root tips, root length, root network area, root average diameter, root volume, and root surface area. These findings indicate that in nitrogen-deficient conditions, maize plants redirect resources to root development and stress tolerance systems, resulting in increased root growth and exploration efficiency. The ON treatment was statistically similar to both the CN and LN treatments across a range of characteristics, suggesting that organic nitrogen is not as effective as chemical nitrogen. Nonetheless, it can support maize growth and development in low-nitrogen environments.
Overall, these findings illustrate the importance of nitrogen management in maize production, emphasising the major impact of nitrogen supply and concentration on plant growth, root development, and physiological processes. These findings have implications for agricultural practices aimed at improving crop yield and sustainability in nitrogen-limited areas. More research in this field is needed to understand the molecular mechanisms behind nitrogen-mediated responses in maize and other crop species, allowing for the creation of novel breeding techniques for increased NUE and stress resilience.
Author Contributions: Conceptualization: SMAR, TAK; Methodology: SMAR, BHM; Formal Analysis: SMAR, RRT; Investigation: SMAR, TAK; Resources: MRI, MNY; Data Curation: SMAR, BB; Writing – Original Draft: SMAR; Writing – Review & Editing: MRI, TAK; Supervision: MRI, TAK. All authors declare that they have read and approved the publication of the manuscript in this present form.
Funding: This research was supported by grants from the Faculty of Agriculture at the University of Rajshahi, Bangladesh.
Acknowledgments: The authors expresses their gratitude to all members of the Farming Systems Engineering Laboratory, Department of Agronomy and Agricultural Extension, University of Rajshahi, Bangladesh.
Conflicts of Interest: Author declare no conflict of interest.
REFERENCES
Anas, M.; Liao, F.; Verma, K.K.; Sarwar, M.A.; Mahmood, A.; Chen, Z.L.; Li, Y.R. Fate of nitrogen in agriculture and environment: agronomic, eco-physiological and molecular approaches to improve nitrogen use efficiency. Biological Research. 2020, 53, 1-20. http://dx.doi.org/10.1186/s40659-020-00312-4
AOAC. Official Methods of Analysis, Association of Official Analytical Chemists, Washington DC, 15th ed., 1990.
Ayyar, S.; Appavoo, S.; Basker, M.; Pandiyarajan, P.; Kavimani, R. Effect of Zinc and microbial inoculation on soil enzyme activities for maize (Zea mays L.) in black soil. International Journal of Current Microbiology and Applied Sciences. 2019, 8, 1804-1814. https://doi.org/10.20546/ijcmas.2019.808.213
Bates, L.S.; Waldren, R.A.; Teare, I.D. Rapid determination of free proline for water-stress studies. Plant and soil. 1973, 39, 205-207. http://dx.doi.org/10.1007/BF00018060
Baweja, P.; Kumar, S.; Kumar, G. Fertilizers and pesticides: Their impact on soil health and environment. Soil Health. 2020, 265-285. http://dx.doi.org/10.1007/978-3-030-44364-1_15
Begam, A.; Ray, M.; Roy, D.C.; Sujit, A. Performance of hybrid maize (Zea mays L.) in different levels and times of nitrogen application in Indo Gangetic plains of eastern India. Journal of Experimental. Biology and Agricultural Sciences. 2018, 6, 929-935. http://dx.doi.org/10.18006/2018.6(6).929.935
Bhattacharya, A. Plant growth hormones in plants under low-temperature stress: A Review. In Physiological processes in plants under low temperature stress. Springer, Singapore, 2022, 517-627.
Briat, J.F.; Gojon, A.; Plassard, C.; Rouached, H.; Lemaire, G. Reappraisal of the central role of soil nutrient availability in nutrient management in light of recent advances in plant nutrition at crop and molecular levels. European Journal of Agronomy. 2020, 116. https://doi.org/10.1016/j.eja.2020.126069
Chen, G.; Wu, P.; Wang, J.; Zhou, Y.; Ren, L.; Cai, T.; Jia, Z. How do different fertilization depths affect the growth, yield, and nitrogen use efficiency in rain-fed summer maize? Field Crops Research. 2023, 290. https://doi.org/10.1016/j.fcr.2022.108759
Chieb, M.; Gachomo, E.W. The role of plant growth promoting rhizobacteria in plant drought stress responses. BMC Plant Biology. 2023, 23, 407. https://doi.org/10.1186/s12870-023-04403-8
Croft, H.; Arabian, J.; Chen, J.M.; Shang, J.; Liu, J. Mapping within-field leaf chlorophyll content in agricultural crops for nitrogen management using Landsat-8 imagery. Precision Agriculture. 2020, 21, 856-880. https://doi.org/10.1007/s11119-019-09698-y
Dao, J.; Xing, Y.; Chen, C.; Chen, M.; Wang, Z. Adaptation of rhizosphere bacterial communities of drought-resistant sugarcane varieties under different degrees of drought stress. Microbiology Spectrum. 2023. https://doi.org/10.1128/spectrum.01184-23
Fan, P.; Ming, B.; Anten, N.P.; Evers, J.B.; Li, Y.; Li, S.; Xie, R. Plastic response of leaf traits to N deficiency in field-grown maize. AoB Plants. 2022, 14. https://doi.org/10.1093/aobpla/plac053
Fathi, A.; Zeidali, E. Conservation tillage and nitrogen fertilizer: a review of corn growth and yield and weed management. Central Asian Journal of Plant Science Innovation. 2021, 1, 121-142. https://doi.org/10.22034/CAJPSI.2021.03.01
Govindasamy, P.; Muthusamy, S.K.; Bagavathiannan, M.; Mowrer, J.; Jagannadham, P.T.K.; Maity, A.; Tiwari, G. Nitrogen use efficiency-a key to enhance crop productivity under a changing climate. Frontiers in Plant Science. 2023, 14. https://doi.org/10.3389%2Ffpls.2023.1121073
Gu, J. Optimizing Irrigation and Nitrogen Regimes in Rice Plants Can Contribute to Achieving Sustainable Rice Productivity. Agronomy. 2023, 13, 2495. https://doi.org/10.3390/agronomy13102495
Hasibuzzaman, A.S.M.; Akter, F.; Bagum, S.A.; Hossain, N.; Akter, T.; Uddin, M.S. Morpho-Physiological Mechanisms of Maize for Drought Tolerance. In Plant Stress Physiology, IntechOpen, London, UK, 2021, pp. 229.
Hemati, A.; Moghiseh, E.; Amirifar, A.; Mofidi-Chelan, M.; Asgari Lajayer, B. Physiological effects of drought stress in plants. In Plant Stress Mitigators: Action and Application, Springer Nature, Singapore, 2022, pp. 113-124.
Hui, J.; An, X.; Li, Z.; Neuhäuser, B.; Ludewig, U.; Wu, X.; Yuan, L. The mycorrhiza-specific ammonium transporter ZmAMT3; 1 mediates mycorrhiza-dependent nitrogen uptake in maize roots. The Plant Cell. 2022, 34, 4066-4087. https://doi.org/10.1093/plcell/koac225
Islam, M.R.; Hoshain, S. A brief review on the present status, problems and prospects of maize production in Bangladesh. Research in Agriculture Livestock and Fisheries. 2022, 9, 89-96. http://dx.doi.org/10.3329/ralf.v9i2.61613
Khan, T.A.; Nadeem, F.; Chen, L.; Wang, X.; Zeng, Z.; Hu, Y. Enhancing naked oat (Avena nuda L.) productivity with minimal indirect nitrogen loss and maximum nitrogen use efficiency through integrated use of different nitrogen sources. PloS ONE. 2019, 14. https://doi.org/10.1371/journal.pone.0213808
Khan, T.A.; Nadeem, F.; Gao, Y.; Yang, Y.; Wang, X.; Zeng, Z.; Hu, Y. A larger root system in oat (Avena nuda L.) is coupled with enhanced biomass accumulation and hormonal alterations under low nitrogen. Applied Ecology and Environmental Research. 2019, 17. http://dx.doi.org/10.15666/aeer/1702_46314653
Kong, L.; Xie, Y.; Hu, L.; Si, J.; Wang, Z. Excessive nitrogen application dampens antioxidant capacity and grain filling in wheat as revealed by metabolic and physiological analyses. Scientific Reports. 2017, 7. http://dx.doi.org/10.15666/aeer/1702_46314653
Liu, X.; Man, X.; Chen, M.; Zhao, C.; Liu, C.; Tong, J.; Qu, B. Transgenerational plasticity in morphological characteristics and biomass of the invasive plant Xanthium strumarium. Ecological Applications. 2023. https://doi.org/10.1002/eap.2920
Mosaad, I.S.; Serag, A.H.; Moustafa-Farag, M.; Seadh, A.K. Effect of exogenous proline application on maize yield and the optimum rate of mineral nitrogen under salinity stress. Journal of Plant Nutrition. 2020, 43, 354-370.
Mu, X.; Chen, Y. The physiological response of photosynthesis to nitrogen deficiency. Plant Physiology and Biochemistry. 2021, 158, 76-82. https://doi.org/10.1016/j.plaphy.2020.11.019
Ochieng’, I.O.; Gitari, H.I.; Mochoge, B.; Rezaei-Chiyaneh, E.; Gweyi-Onyango, J.P. Optimizing maize yield, nitrogen efficacy and grain protein content under different N forms and rates. Journal of Soil Science and Plant Nutrition. 2021, 21, 1867-1880. https://doi.org/10.1007/s42729-021-00486-0
Ohyama, T. The role of legume-rhizobium symbiosis in sustainable agriculture. Legume nitrogen fixation in soils with low phosphorus availability: Adaptation and regulatory implication, 1st Edition. Springer Cham, 2017, 1-20.
Pan, X.; Wang, P.; Wei, X.; Zhang, J.; Xu, B.; Chen, Y.; Wang, Z. Exploring root system architecture and anatomical variability in alfalfa (Medicago sativa L.) seedlings. BMC Plant Biology. 2023, 23, 449.
http://dx.doi.org/10.1186/s12870-023-04469-4
Peng, W.A.N.G.; Wang, C.D.; Wang, X.L.; Wu, Y.H.; Zhang, Y.; Sun, Y.G.; Mi, G.H. Increasing nitrogen absorption and assimilation ability under mixed NO3− and NH4+ supply is a driver to promote the growth of maize seedlings. Journal of Integrative Agriculture. 2023, 22, 1896-1908. https://doi.org/10.1016/j.jia.2023.04.037
Rodríguez-Espinosa, T.; Papamichael, I.; Voukkali, I.; Gimeno, A.P.; Candel, M.B.A.; Navarro-Pedreño, J.; Lucas, I.G. Nitrogen management in farming systems under the use of agricultural wastes and circular economy. Science of The Total Environment. 2023, 10. https://doi.org/10.1016/j.scitotenv.2023.162666
Saha, L.; Bauddh, K. Sustainable agricultural approaches for enhanced crop productivity, better soil health, and improved ecosystem services. In Ecological and Practical Applications for Sustainable Agriculture. Springer, Singapore, 2020, 1-23.
Sahoo, S.; Adhikari, S.; Joshi, A.; Singh, N.K. Use of wild progenitor teosinte in maize (Zea mays) improvement: present status and future prospects. Tropical Plant Biology. 2021, 14, 156-179. https://doi.org/10.1007/s12042-021-09288-1
Schneider, H.M.; Yang, J.T.; Brown, K.M.; Lynch, J.P. Nodal root diameter and node number in maize (Zea mays L.) interact to influence plant growth under nitrogen stress. Plant Direct. 2021, 5. https://doi.org/10.1002/pld3.310
Shaji, H.; Chandran, V.; Mathew, L. Organic fertilizers as a route to controlled release of nutrients. Controlled release fertilizers for sustainable agriculture. 2021, 231-245.
Song, Y.; Li, J.; Liu, M.; Meng, Z.; Liu, K.; Sui, N. Nitrogen increases drought tolerance in maize seedlings. Functional Plant Biology. 2019, 46, 350-359. https://doi.org/10.1071/fp18186
Su, W.; Ahmad, S.; Ahmad, I.; Han, Q. Nitrogen fertilization affects maize grain yield by regulating nitrogen uptake, radiation and water use efficiency, photosynthesis, and root distribution. Plant Biology. 2020. https://doi.org/10.7717/peerj.10291
Wang, Y.; Huang, Y.; Fu, W.; Guo, W.; Ren, N.; Zhao, Y.; Ye, Y. Efficient physiological and nutrient use efficiency responses of maize leaves to drought stress under different field nitrogen conditions. Agronomy. 2020, 10, 523. https://doi.org/10.3390/agronomy10040523
Wang, Y.; Zhang, X.; Chen, J.; Chen, A.; Wang, L.; Guo, X.; Gao, Q. Reducing basal nitrogen rate to improve maize seedling growth, water, and nitrogen use efficiencies under drought stress by optimizing root morphology and distribution. Agricultural Water Management. 2019, 212, 328-337. https://doi.org/10.1016/j.agwat.2018.09.010
Wen, B., Xiao, W., Mu, Q., Li, D., Chen, X., Wu, H.; Peng, F. How does nitrate regulate plant senescence? Plant Physiology and Biochemistry. 2020, 157, 60-69. https://doi.org/10.1016/j.plaphy.2020.08.041
Whetton, R.L.; Harty, M.A.; Holden, N.M. Communicating nitrogen loss mechanisms for improving nitrogen use efficiency management, focused on global wheat. Nitrogen. 2022, 3, 213-246. https://doi.org/10.3390/nitrogen3020016
Yang, Z.; Wang, Z.; Yang, C.; Yang, Z.; Li, H.; Wu, Y. Physiological responses and small RNAs changes in maize under nitrogen deficiency and resupply. Genes Genomics. 2019, 41, 1183-1194. https://doi.org/10.1007/s13258-019-00848-0
Zhang, D.; Sun, Z.; Feng, L.; Bai, W.; Yang, N.; Zhang, Z.; Zhang, L. Maize plant density affects yield, growth, and source-sink relationship of crops in maize/peanut intercropping. Field Crops Research. 2020, 257. http://dx.doi.org/10.1016/j.fcr.2020.107926
Zhao, X.; Dong, Q.; Han, Y.; Zhang, K.; Shi, X.; Yang, X.; Yu, H. Maize/peanut intercropping improves nutrient uptake of side-row maize and system microbial community diversity. BMC microbiology. 2022, 22, 14. https://doi.org/10.1186/s12866-021-02425-6
Academic Editor: Dr. Isabela Maria Simion
Publisher Note: Regarding jurisdictional assertions in published maps and institutional affiliations ALSE maintain neutrality.
Biswas Bitopi, Islam M. Robiul, Khan Tariful Alam, Momen Billal Hossain, Rahman S.M. Abidur, Tanvir Rashedur Rahman, Yasmin Mosammat Nilufar