Alina Elena Trofin, Elena Ungureanu, Lucia Carmen Trincă, Maria Emiliana Fortună, Diana Beatrice Eperjessy
ABSTRACT. The adsorption of metal ions from increasing concentrations in aqueous solutions by modified straw lignin Protobind 1000 was studied. The effect of metallic ion concentrations (from 20.72 to 207.2 mg·L-1 for Pb2+ and from 6.538 to 65.38 mg·L-1 for Zn2+) and contact time (30, 60 and 90 minutes) were studied at pH = 6 and 200C. Langmuir and Freundlich isotherm equations were applied to assess equilibrium data and the kinetics of the adsorption processes were analysed using Lagergren pseudo first order and Ho&McKay pseudo second order models. The results show that the adsorption processes reached equilibrium after 90 minutes, but similar values were registered after 60 minutes. The Freundlich isotherm described the process better, denoting chemisorption with the formation of ion-lignin complex structures. The Ho&McKay model fit the adsorption data better with regression coefficients equal to 1 compared to the Lagergren model, where the regression factors varied between 0.72 and 0.95. For the maximum concentration of lead solution and the longest adsorption time of 90 minutes, the Ho&McKay model predicted an equilibrium capacity qe of 13.1406 mg·g-1 compared to the 13.1398 mg·g-1 obtained. For zinc adsorption, the same maximum concentration and time were considered, and the pseudo-second order model predicted a qe of 12.6743 mg·g-1 compared to the obtained value of 12.6714 mg·g-1.
The uptake of lead was greater on 0.15 g of adsorbent (a maximum of 27.23 mg·g-1) than the zinc uptake (a maximum of 8.28 mg·g-1), for all analysed concentrations.
Keywords: adsorption, Protobind 1000 (PB 1000), lead, zinc.
Cite
ALSE and ACS Style
Trofin, A.E.; Ungureanu, E.; Trincă, L.C.; Fortună, M.E.; Eperjessy, D.B. Potential valorisation of Protobind 1000 as adsorbent for Pb2+ and Zn2+. Journal of Applied Life Sciences and Environment 2022, 55(1), 31-44. https://doi.org/10.46909/alse-551044
AMA Style
Trofin AE, Ungureanu E, Trincă LC, Fortună ME, Eperjessy DB. Potential valorisation of Protobind 1000 as adsorbent for Pb2+ and Zn2+. Journal of Applied Life Sciences and Environment. 2022; 55(1): 31-44. https://doi.org/10.46909/alse-551044
Chicago/Turabian Style
Trofin, Alina, Elena, Elena Ungureanu, Lucia Carmen Trincă, Maria Emiliana Fortună, and Diana Beatrice Eperjessy. 2022. “Potential valorisation of Protobind 1000 as adsorbent for Pb2+ and Zn2+” Journal of Applied Life Sciences and Environment 55, no. 1: 31-44. https://doi.org/10.46909/alse-551044
View full article (HTML)
Potential Valorisation of Protobind 1000 as Adsorbent for Pb2+ and Zn2+
Alina Elena TROFIN1,*, Elena UNGUREANU1, Lucia Carmen TRINCĂ1, Maria Emiliana FORTUNĂ2 and Diana Beatrice EPERJESSY3
1 “Ion Ionescu de la Brad” University of Life Sciences, Faculty of Horticulture, Department of Exact Sciences, 3, Mihail Sadoveanu Alley, 700490, Iasi, Romania; email: eungureanu@uaiasi.ro, lctrinca@uaiasi.ro
2 “Petru Poni” Institute of Macromolecular Chemistry, 41A, Grigore Ghica Voda Al., 700487, Iasi, Romania; email: fortuna.maria@icmpp.ro
3 “Saint Mary” Emergency Children Hospital, 62, Vasile Lupu Str., 700309, Iasi, Romania; email: diana.eperjessy@gmail.com
*Correspondence: atrofin@uaiasi.ro
Received: May 5, 2022. Revised: May 25, 2022. Accepted: June 4, 2022. Published online: Oct. 25, 2022
ABSTRACT. The adsorption of metal ions from increasing concentrations in aqueous solutions by modified straw lignin Protobind 1000 was studied. The effect of metallic ion concentrations (from 20.72 to 207.2 mg·L-1 for Pb2+ and from 6.538 to 65.38 mg·L-1 for Zn2+) and contact time (30, 60 and 90 minutes) were studied at pH = 6 and 200C. Langmuir and Freundlich isotherm equations were applied to assess equilibrium data and the kinetics of the adsorption processes were analysed using Lagergren pseudo first order and Ho&McKay pseudo second order models. The results show that the adsorption processes reached equilibrium after 90 minutes, but similar values were registered after 60 minutes. The Freundlich isotherm described the process better, denoting chemisorption with the formation of ion-lignin complex structures. The Ho&McKay model fit the adsorption data better with regression coefficients equal to 1 compared to the Lagergren model, where the regression factors varied between 0.72 and 0.95. For the maximum concentration of lead solution and the longest adsorption time of 90 minutes, the Ho&McKay model predicted an equilibrium capacity qe of 13.1406 mg·g-1 compared to the 13.1398 mg·g-1 obtained. For zinc adsorption, the same maximum concentration and time were considered, and the pseudo-second order model predicted a qe of 12.6743 mg·g-1 compared to the obtained value of 12.6714 mg·g-1.
The uptake of lead was greater on 0.15 g of adsorbent (a maximum of 27.23 mg·g-1) than the zinc uptake (a maximum of 8.28 mg·g-1), for all analysed concentrations.
Keywords: adsorption, Protobind 1000 (PB 1000), lead, zinc.
INTRODUCTION
Heavy metals are known to cause severe damages to animal organisms (Rao et al., 2011). Different methods are used for their removal (ion exchange, precipitation, separation, or reverse osmosis) (Amer et al., 2010; Saleh 2021), but they are expensive or inefficient at low concentrations. Therefore, adsorption is usually preferred because it is efficient, easy to use and different adsorbents are available (activated carbon, zeolites, clay minerals or resins) (Rao et al., 2011; Saleh, 2021). Efforts have been taken to use phytoremediation (Bello et al., 2018) or to obtain low-cost adsorbents, nanomaterials, polymers, and green materials to remove metals from waste waters of household or industrial origin (Esmaili et al., 2003; Janyasuthiwong et al., 2015; Kurniawan et al., 2006; Saleh, 2016, 2021; Sani et al., 2017; Al Hamouz et al., 2017).
The highest amount of lead acceptable in water is 0.05-0.1 mg·L-1 and for zinc, 5 mg·L-1. These ions are not biodegradable and can accumulate in plants and animals, causing multiple effects (Depci et al., 2012). Studies have been made on the ability of plant or animal-based biochar to retain heavy metal ions. This is due to the hydroxyapatite content of biochar(Xue et al., 2020; Sellaoui et al., 2019; Xu et al., 2013; Meng et al., 2018; Trakal et al., 2011).
The objective of this study is to determine if lead and zinc at different concentrations in aqueous solutions can be retained on thermally treated Protobind 1000.
MATERIALS AND METHODS
Material and reagents
Protobind 1000 (PB 1000) lignin derivative is a commercial product supplied by Granit Recherche Développement S.A. from Lausanne, Switzerland. It is residual lignin separated by the process of alkaline descaling of some annual plants (cereal straws such as wheat or barley). It is heat-treated at 70 ºC in order to increase its stability to the action of environmental factors. The properties and an SEM image of the surface of this modified lignin are presented in Table 1 and Figure 1 (Gîlcă et al., 2013)
Lignin is an amorphous natural resin with a three-dimensional aromatic polymer structure bearing various functional groups (alkyl and aromatic hydroxylic groups, carbonyl, methoxy etc.) These functional groups are capable of forming bonds with metallic ions and therefore have ion-exchange ability. This makes lignin a potentially useful adsorbent material for retaining heavy metals from different solutions.
Table 1
Protobind 1000 properties
Properties |
|
Relative humidity, % |
4.2 |
Ash, % |
1.4 – 1.8 |
Solubility in acids % |
1,2 |
Insolubility in acids % |
91.5 |
Solubility in aqueous alkali % |
94 |
Solubility in furfuryl alcohol, % |
40.1 |
T softening, °C |
200 |
pH (10% aqueous suspension) |
3.5 |
Particle size |
>99% <210 mm |
Density (g/cm3) |
0.3 |
Aryl OH, mmoles / g |
1.8 – 1.9 |
COOH, mmoles / g |
2.1 – 2.3 |
Table 2 contains the distribution of PB 1000 functional groups.
Table 2
Functional groups content of Protobind 1000
T0C |
– |
90 |
90 |
50 |
50 |
pH |
– |
12.0 |
10.5 |
10.5 |
12.0 |
OH total groups |
1.11 |
1.23 |
1.15 |
1.14 |
1.16 |
Ar-OH groups |
0.89 |
0.98 |
0.98 |
0.98 |
0.99 |
OCH3 groups |
1.05 |
1.15 |
1.13 |
1.12 |
1.14 |
Alk/Ar ratio |
1.17 |
1.27 |
1.20 |
1.22 |
1.21 |
C=O groups |
0.89 |
0.95 |
0.91 |
0.95 |
0.94 |
Siringyl/ Guaiacyl ratio |
0.83 |
0.96 |
0.96 |
0.96 |
0.96 |
Thermogravimetry is well-known and it is often used to analyse lignin’s thermodynamic properties. Table 3 shows the decomposition temperature of PB 1000.
Table 3
Characteristics of Protobind 1000 thermal degradation process
Stage |
I |
II |
III |
Ti (°C) |
52 |
229 |
330 |
Tmax (°C) |
77 |
267 |
383 |
Tf (°C) |
106 |
330 |
532 |
W (%) |
3.31 |
17.05 |
42.47 |
Ti – initial temperature – degradation starts; Tmax – temperature at the maximum rate of degradation, Tf – final temperature and W – mass loss %.
Among spectral analysis techniques, nuclear magnetic resonance (NMR) provides the most complex structural information for organic compounds. For the 1H NMR spectroscopic characterization, shown in Figure 2, PB 1000 was first acetylated to ease dissolution in hexa deuterium dimethyl sulfoxide (DMSO-D6) (Ungureanu et al., 2016).
For the initial solutions used in the experiment, concentrations in the range of 20.72 – 207.2 mg·L-1 for Pb2+ ions and in the range 6.538 – 65.38 mg·L-1 for Zn2+ ions were chosen. From each solution a volume of 20 mL was measured and mixed with 0.15 g of adsorbent (modified lignin PB 1000). The contact intervals of time selected after the preliminary tests were 30, 60 and 90 minutes, at a temperature of 200C, considering that the influence of temperature variation (± 5 0C from the considered one) on the adsorption on lignin varieties is insignificant, according to Guo et al. (2008).
The adsorption was performed in a dynamic procedure, at 120 rotations per minute, followed by separation of the phases by filtration on Whatman quantitative filter paper.
The determination of Pb2+ concentration was performed spectrophotometrically, using PAR (4- (2-pyridylazo) – resorcinol), in alkaline medium (pH = 10, ammonia buffer) as a colouring reagent that forms a red-orange complex with a maximum absorption at 530 nm.
Reagents Pb
10-2 M PAR reagent (dissolve 0.273 g of monosodium PAR into 100 ml);
Buffer solution (pH 10). A mixture of 85 ml NH4OH and 26 g NH4Cl was used to insure a pH of 10 in 1 L of solution.
10 ml Pb solution, 15 ml buffer and 1 ml PAR are mixed and shaken for 30 seconds and then brought to 50 ml with distilled water in a volumetric flask.
Samples could be analysed immediately or up to 24 hours later (Dagnall et al., 1965).
The spectrophotometric determination of Zn2+ was performed using xylenol orange, which forms a red complex with Zn2 + ions, with a maximum absorption at 570 nm.
Zn
Stock xylenol orange solution (1.58·10-3 M in deionized water);
Buffer solution (pH = 6.00) was prepared using KH2PO4 (0.1 M) + Na2B4O7·10H2O (0.05 M).
In a 10 mL volumetric flask we measured 5 mL Zn solution and added 2 mL of 1.58·10-3 M xylenol orange solution; the flask was filled to the mark with buffer solution and mixed (Tehrani et al., 2012).
Instruments
The pH values for the initial samples were determined with an analog pH-meter / ORP-meter HI83141 from Hanna Instruments and were established at pH 6 to ensure a higher efficiency of adsorption (in terms of enabling the dissociation for the surface functional groups) and not to exceed the values at which precipitations of the considered metal ions can take place (pH values > 6.5), according to Chen et al., (2008) and Erdem et al., (2013).
A VIS spectrophotometer V1000 SN: YA07151909217 and glass vats with a 1 cm optical path were used for the spectrophotometric measurement of the solutions resulting from the adsorption.
RESULTS AND DISCUSSION
Thermodynamic studies
1. Freundlich isotherm
The Freundlich isotherm model usually applies to sorption processes on heterogeneous surfaces and also to multilayer sorption. It is expressed by the following equation (Eq. 1):
qe = kF Ce 1/n (1),
where n and kF are the Freundlich constants.
We applied the linear form of the Freundlich isotherm (Eq. 2):
log 𝑞𝑒 = log k𝐹 + 1/𝑛 log𝐶𝑒 (2),
where qe is the adsorption capacity (mg·g-1), 𝐶𝑒 is the concentration at equilibrium (mg·L-1) and k𝐹 and 1/n are Freundlich constants (Ayawei et. al., 2017).
Experimental data complying with a Freundlich isotherm indicates the heterogeneity of the PB 1000 surface. The value of the 1/n factor shows the adequacy and efficiency of the adsorbent/adsorbate system. The linear plot of ln qe versus ln Ce (presented in Figure 3 for Pb2+ and in Figure 4 for Zn2+ after 60 minutes adsorption time) shows that the adsorption fits the Freundlich model.
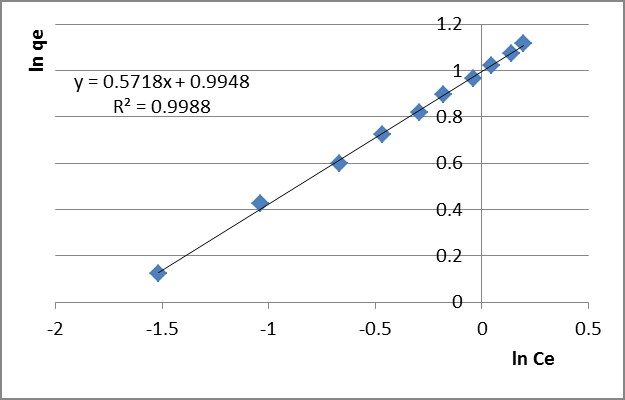
Figure 3 – Linear Freundlich isotherm for Pb2+ adsorption after 60 minutes
kF and 1/n were determined and presented in Table 4.
2. Langmuir isotherm
Langmuir adsorption serves to quantify the adsorption capacity of various adsorbents. The following equation was applied (Eq. 3):
qe = kL qmax Ce/(1 + qmax Ce) (3),
The Langmuir constants kL and qmax relate to the adsorption capacity and energy. The linear form of the Langmuir equation we used to calculate the specific parameters is as follows (Eq. 4):
1/qe = 1/(kL·qmax·Ce) + 1/qmax (4),
where qe is concentration of adsorbate at equilibrium (mg·g-1), qmax is the maximum sorption capacity (mg·g-1) and Ce is the equilibrium concentration in mg·L-1.
The characteristics of the Langmuir isotherm are expressed by the separation factor RL (Eq. 5):
RL= 1/(1+kL C0) (5),
where kL is the Langmuir constant (mg·g−1) and C0 represents the initial concentration of ions (mg·L−1). RL values indicate the process to be unfavourable when RL > 1, linear when RL =1, favourable when 0< RL < 1, and irreversible when RL = 0. (Cara et al., 2016)
The variation of 1/qe versus 1/Ce was applied for the selected time intervals (presented in Figure 5 for Pb2+ and in Figure 6 for Zn2+ after the 60 min adsorption time). kL, qmax and RL were determined from the Langmuir isotherm plots and they are presented in Table 4.
The experimental data fit with the Freundlich isotherm model better. This proves that chemisorption is involved in retaining the lead and zinc ions, resulting complex structures with the functional groups on the sorbent’s active sites.
Kinetic modelling
We applied two kinetic models to assess the experimental data:
(a) Lagergren (pseudo first order) model, characterized by the following linear equation (Eq. 6):
ln(qe − qt) = ln qe − k1·t (6),
where k1 represents the rate constant of this model (min-1).
(b) Ho and McKay (pseudo second order) model, expressed in the following linear form (Eq. 7):
t/qe = 1/k2·qe2 + 1/qe (7),
where k2 is the pseudo-second order (mg g-1 min-1) rate constant.
For both models, qe is the quantity of retained ion (mg g-1) at equilibrium and qt is the quantity of retained ion (mg g-1) at a certain time t (Hanif et al., 2017).
The linear plot of t(min) versus ln(qe − qt) in the Lagergren model was used to calculate qe, k1 and R2 for each initial concentration of the two considered ions. For the Ho and McKay model, the linear plot of t(min) versus t/qt was used to determine the characteristic parameters qe, k1 and R2. All of the data obtained are presented in Table 5.
Table 4
Isothermal parameters determined by adsorption of Pb2+ and Zn2+ ions on PB 1000 modified lignin
Time (min) |
Freundlich isotherm |
Langmuir isotherm |
|||||
1/n |
kF |
R2 |
qmax |
kL |
RL |
R2 |
|
Pb2+ adsorption |
|||||||
30 |
0.648 |
2.574 |
0.9906 |
9.634 |
0.103 |
0.045 |
0.9429 |
60 |
0.572 |
2.704 |
0.9988 |
9.852 |
0.101 |
0.045 |
0.9735 |
90 |
0.643 |
1.922 |
0.996 |
11.074 |
0.089 |
0.051 |
0.9741 |
|
Zn2+ adsorption |
||||||
30 |
0.659 |
1.722 |
0.9956 |
10.225 |
0.096 |
0.137 |
0.9609 |
60 |
0.635 |
1.874 |
0.9967 |
11.050 |
0.089 |
0.146 |
0.9765 |
90 |
0.643 |
1.922 |
0.996 |
11.050 |
0.089 |
0.146 |
0.9765 |
Analysing the kinetic parameters, the pseudo-second order model describes better than the pseudo-first order model the adsorption process of both lead and zinc ions, with very small differences between the estimated (qe) and realized (qt) adsorption capacities, as seen in Table 5.
Regarding the efficiency of the lead and zinc ions’ adsorption on modified PB 1000 lignin, we present in Table 6 the quantities of ions retained on the adsorbent depending on the contact time and initial concentration.
Lead retention on lignin-based materials takes place during two steps of the sorption mechanism. In the first step, a quite fast adsorption occurs at the surface, and a slower step, involving intra particle diffusion follows. The Pb2+ binding to the adsorbent‘s active sites takes place by ion exchanges in the first step and then, the diffusion of Pb2+ into the pores occurs in the second step (Bulgariu et al., 2016).
A study comprising 102 publications during 1984 – 2005 showed that cheap adsorbents obtained from agricultural waste proved to be very efficient in removing heavy metal ions (Cr6+ – 170 mg/g on hazelnut shell ash, Ni2+ – 158 mg/g on orange peel ash, Cu2+ – 154.9 mg/g on soybean hull activated with NaOH and citric acid, Cd2+ – 52.08 mg g−1 on jackfruit ash) compared to activated carbon (Cd2+ – 146 mg g−1, Cr6+ – 145 mg g−1, Cr3+ – 30 mg g−1, Zn2+ – 20 mg g−1). Therefore, these materials may prove to be useful alternatives to activated carbon in contaminated water treatment procedures (Kurniawan et al., 2006).
Due to its structure and capability of retaining heavy metal ions, lignin (Figure 7, Ruthran et al., 2021) may be added to the list of alternative adsorbent materials, as previous studies have shown (Nacu et al., 2015; Bulgariu et al, 2016). Lignin – based adsorbents can have great advantages for the environment because they are biocompatible, stable and abundant in nature (Ge et al., 2018).
However, the capacity of lignin materials to retain pollutants is lower than the commercial adsorbents and the resin materials’ one. Composite lignin-based materials with added functional groups were created in order to increase the sorption capacity of lignin (Ruthran et al., 2021).
Usually, chemical modification is applied to improve the reactivity and enrich the functionality of lignin for valorisation.
Recent progress in studying lignin and its derivatives stated that chemical improvements might very well stabilise and enrich the lignin surface to make it a viable wastewater treatment agent, next to other adsorbents, flocculants and sterilants (Wang et al., 2021).
To obtain an efficient adsorbent for the retention of Pb2+, Cd2+ and Ni2+, Ponomarev et al., (2019) developed a nanocomposite using hydrolytic lignin and magnesium hydroxide. Also, they considered regeneration after metal removal. The nanocomposite proved that ion exchanges occurred between all three retained metals and the magnesium – lignin material.
Lin (2022) developed an enhanced lignin adsorbent by demethylation and introducing phenol and amine groups from γ-polyglutamic acid and ε-poly-L-lysine, respectively. These modified lignin materials had higher reactivity and a larger number of surface active sites. They were tested and good results were obtained for removing heavy metals from aqueous solutions. The kinetic and isothermal aspects of the adsorption showed that a pseudo-second-order model and the Langmuir model described both processes better.
Table 5
Parameters determined from kinetic models for adsorption of Pb2+ and Zn2+ ions on modified lignin PB 1000
Initial conc. (mg/L) |
Lagergren pseudo-first order |
Ho & McKay pseudo-second order |
||||||
qe |
k1 |
R2 |
qe |
qt |
k2 |
R2 |
||
Pb2+ adsorption |
||||||||
20.72 |
0.7378 |
-0.0015 |
0.9347 |
1.3307 |
1.3306 |
20.7630 |
1 |
|
41.44 |
2.3284 |
-0.0019 |
0.9214 |
2.6567 |
2.6558 |
5.6898 |
1 |
|
62.16 |
5.4173 |
-0.0022 |
0.8600 |
3.9746 |
3.9733 |
4.0065 |
1 |
|
82.88 |
3.1689 |
-0.0019 |
0.8913 |
5.2910 |
5.2902 |
3.9690 |
1 |
|
103.6 |
2.4552 |
-0.0017 |
0.8840 |
6.6050 |
6.6038 |
3.8203 |
1 |
|
124.32 |
6.1306 |
-0.0022 |
0.8268 |
7.9177 |
7.9164 |
3.3233 |
1 |
|
145.04 |
2.8974 |
-0.0017 |
0.8661 |
9.2251 |
9.2199 |
2.5001 |
1 |
|
165.76 |
4.2224 |
-0.0018 |
0.8229 |
10.5374 |
10.5315 |
2.1443 |
1 |
|
186.48 |
3.9531 |
-0.0018 |
0.8249 |
11.8343 |
11.8289 |
2.1001 |
1 |
|
207.2 |
0.7343 |
-0.0008 |
0.9550 |
13.1406 |
13.1398 |
1.9304 |
1 |
|
Zn2+ adsorption |
||||||||
6.538 |
1.3134 |
-0.0017 |
0.9312 |
1.3142 |
1.3138 |
9.9308 |
1 |
|
13.076 |
3.3248 |
-0.0018 |
0.8844 |
2.6055 |
2.6028 |
2.4428 |
1 |
|
19.614 |
6.6048 |
-0.002 |
0.8331 |
3.9108 |
3.9063 |
1.1696 |
1 |
|
26.152 |
7.5595 |
-0.002 |
0.7969 |
5.1840 |
5.1792 |
1.0279 |
1 |
|
32.69 |
3.07499 |
-0.0014 |
0.8607 |
6.4185 |
6.4148 |
0.8370 |
1 |
|
39.228 |
6.7619 |
-0.0018 |
0.7887 |
7.6923 |
7.6854 |
0.5868 |
1 |
|
45.766 |
10.2267 |
-0.0019 |
0.7674 |
8.9525 |
8.9411 |
0.4159 |
0.9999 |
|
52.304 |
7.6492 |
-0.0017 |
0.7637 |
10.2041 |
10.1939 |
0.3904 |
1 |
|
58.842 |
7.8170 |
-0.0017 |
0.746 |
11.4548 |
11.4456 |
0.3496 |
1 |
|
65.38 |
7.8995 |
-0.0017 |
0.7469 |
12.6743 |
12.6714 |
0.3773 |
1 |
The highest uptakes for Pb2+ (following esterification of lignin materials with carbon sulphide) were 275.9 mg g−1 and 231.8 mg g−1 at 250C. The added functional groups, like amino, hydroxyl, dithiocarbamate or methoxyl formed bonds with Pb2+.
Another method of lignin modification lead to phosphorylated alkali lignin microparticles. Their adsorption capacity for Pb2+ reached 349.2 mg·g-1, as reported by Gong et al. (2021).
Song et al., (2018) tested the Pb2+ removal capacity (the uptake per unit mass) of activated carbon adsorbents obtained from waste materials and observed a decrease in their efficiency when the amounts of the adsorbent increased. This is due to lower adsorbate / adsorbent ratio. Therefore, 0.1 g of adsorbent was used for all experiments. With regard to the adsorption conditions, the temperature varied between 25 – 45 °C and the pH was 7, but the results proved that temperature had no visible effect on Pb2+ uptake. Also, the influence of adsorption time (ranging from 10 to 60 min) on Pb2+ uptake was tested,. The adsorption efficiency of activated carbon materials obtained from sawdust, acrylic fabric, tire powder and rice husk tended to stabilize at longer adsorption time, achieving equilibrium after 60 min. The maximum Pb2+ uptake for the four studied adsorbents was 58.25 mg·g-1, 31.25 mg·g-1, 50.48 mg·g-1 and 59.73 mg·g-1, respectively.
Table 6
Quantities of Pb2+ and Zn2+ adsorbed on modified lignin PB 1000
Initial conc.( mg·L-1) |
30 min |
60 min |
90 min |
Pb2+ adsorption |
|||
20.72 |
2.7502 |
2.7542 |
2.7570 |
41.44 |
5.4741 |
5.5000 |
5.5028 |
62.16 |
8.1921 |
8.2283 |
8.2327 |
82.88 |
10.9203 |
10.9563 |
10.9613 |
103.6 |
13.6427 |
13.673 |
13.683 |
124.32 |
16.3563 |
16.3925 |
16.4029 |
145.04 |
19.0424 |
19.0874 |
19.1036 |
165.76 |
21.7541 |
21.796 |
21.8213 |
186.48 |
24.4402 |
24.4824 |
24.5096 |
207.2 |
27.1535 |
27.1938 |
27.2257 |
Zn2+ adsorption |
|||
6.538 |
0.8541 |
0.8578 |
0.8589 |
13.076 |
1.6810 |
1.6991 |
1.7017 |
19.614 |
2.5107 |
2.5490 |
2.5539 |
26.152 |
3.3392 |
3.3756 |
3.3862 |
32.69 |
4.1361 |
4.1816 |
4.1940 |
39.228 |
4.9431 |
5.0052 |
5.0247 |
45.766 |
5.7288 |
5.8235 |
5.8457 |
52.304 |
6.5448 |
6.6309 |
6.6648 |
58.842 |
7.3542 |
7.4346 |
7.4831 |
65.38 |
8.1672 |
8.2345 |
8.2846 |
Amer et al., (2011) found that the sorption capacity on sodium polyphosphate kaolinite clay was 40.00 mg g−1 for Pb2+ and f, 27.78 mg g−1 or Zn2+.
Chen et al., (2008) found that the maximum biosorption capacity for Pb2+ by waste brewery biomass was 0.413 mmol Pb2+·g-1.
Another study on Pb2+ and Zn2+ removal showed that the adsorption capacity of lead was 15.56 mg·g-1 and the one for zinc was 11.72 mg·g-1 on activated carbon derived from Van apple pulp. A film diffusion process was responsible for the adsorption, which proved to be endothermic, feasible and thermodynamically favoured. Depci et al. (2012) and Erdem et al. (2013) found that the maximum monolayer adsorption capacity of activated carbon obtain from soybean oil cake activated with K2CO3 was 476.2 mg·g-1. From the kinetics point of view, the Ho and McKay model best suited the experimental data and the thermodynamic study showed the adsorption process to be spontaneous and endothermic.
Adsorption mechanism
There are five mechanisms proposed for metal retention from water on different adsorbents. They are the following: – electrostatic interactions between metallic ions and active sites on the surface; – cation exchange between metallic ions and other cations on the surface, including protons; – complexes formed with the functional groups of the sorbent’s structure; – precipitation of the metallic ions and – reduction and sorption of the reduced species on the active sites. The adsorption mechanisms and the amount of metal adsorbed vary with the surface properties, adsorption conditions (as pH) and the selected metals.
The highest adsorbed amounts were observed at pH 6.0 (provided by acetate buffer), when over 96% of Pb2+ was removed from solution. The competition between Pb2+ and H3O+ for the active sites of the surface is mainly responsible for the process. The strong pH-dependence of the adsorption process suggests that the specific adsorption mechanism may be explained by electrostatic interactions between metallic cations and negatively charged functional groups.
The metal ion biosorption on lignin takes place through sequential equilibrium steps: first, very fast biosorption at the surface and second a slower intra-particle diffusion. Ion exchanges in the first step are responsible for retaining cations on the surface, while their diffusion into the adsorbent’s pores occurs effectively in the second step. The fact that the retention of Pb2+ on the surface of lignin-based adsorbents takes place mainly by electrostatic interactions (ion-exchange or complexation) is confirmed by the short contact time needed to reach equilibrium.
The pH greatly affects the adsorbents’ capacity for Pb2+ removal. The efficiency increases with the increase of pH, because at lower values, the amount of H3O+ that can compete for the oxygen – containing functional groups is larger, therefore reducing the possibility of Pb2+ ions to occupy active sites on the surface. When pH increases, the active sites become available again for the metallic ions, leading to higher amounts retained per mass unit (Bulgariu et al., 2013).
Ungureanu et al. (2021) showed that the adsorption of Pb2+ and Zn2+ ions from aqueous solutions on unmodified Sarkanda grass lignin probably occurs in two successive stages: – by ion-exchange surface interactions followed by ion retention on the functional groups of lignin which takes place through intraparticle diffusion.
CONCLUSION
As an alternative to activated carbon and other expensive adsorbents, PB 1000 modified lignin can retain heavy metal ions from aqueous solutions, even when the concentrations of the ions are low.
The adsorption processes for both lead and zinc were best described by the Freundlich isotherm, allowing us to conclude that chemisorption occurred with the formation of ion-lignin complex structures. The kinetics of the adsorption process were best fitted by the pseudo-second order Ho&McKay model, with regression coefficients equal to 1. Considering the maximum concentration of lead solution applied and the longest adsorption time of 90 minutes, the pseudo second order model predicted an adsorption capacity qe of 13.1406 mg·g-1 compared to the 13.1398 mg·g-1 obtained. For the zinc adsorption, the Ho and McKay model predicted a qe of 12.6743 mg·g-1 compared to the obtained value of 12.6714 mg·g-1.
The uptake of lead on the Protobind 1000 lignin reached a maximum of 27.23 mg·g-1 which is greater than the zinc uptake (a maximum of 8.28 mg·g-1).
Considering the fact that the adsorption takes place with good results after one hour, using small quantities (5 to 7.5 g·L-1) and minimum conditioning, modified lignin PB 1000 can be taken into consideration as a promising material for further studies on activation and surface enhancement in order to increase its sorption potential.
Author Contributions: Conceptualization A.T., E.U., L.C.T; methodology A.T., M.E.F, L.C.T; analysis M.E.F, A.T., E.U., investigation A.T., M.E.F, E.U., resources E.U., A.T., M.E.F., data curation A.T., D.B.T., writing D.B.T, A.T., review A.T., L.C.T., D.B.T., supervision A.T., E.U. All authors have read and agreed to the published version of the manuscript.
Funding: There was no external funding for this study.
Acknowledgement: The authors would like to thank Granit Recherche Développement S.A., for supplying the lignin samples, in the framework of the ECOBINDERS program (SIXTH FRAMEWORK PROGRAMME, NMP2-CT-2005-011734).
Conflicts of Interest: The authors declare no conflict of interest.
REFERENCES
Al Hamouz, O.C.S.; Adelabu, I.O.; Saleh, T.A. (2017). Novel cross-linked melamine based polyamine/CNT composites for lead ions removal, Journal of Environmental Management. 192, 163-170. https://doi.org/10.1016/ j.jenv man.2017.01.056.
Amer, M.W.; Khalili, F.I.; Awwad, A.M. (2010). Adsorption of lead, zinc and cadmium ions on polyphosphate-modified kaolinite clay. Journal of Environmental Chemistry and Ecotoxicology. 2(1), 1-8. https://doi.org/ 10.5897/JEC E.9000005.
Ayawei, N.; Ebelegi, A.N.; Wankasi, D. (2017). Modelling and Interpretation of Adsorption Isotherms. Journal of Chemistry. https://doi.org/ 10.1155/2017/3039817.
Bello, A.O.; Tawabini, B.S.; Khalil, A.B.; Boland, C.R.; Saleh, T.A. (2018). Phytoremediation of cadmium-, lead- and nickel-contaminated water by Phragmites australis in hydroponic systems. Ecological Engineering. 120, 126-133, https://doi.org/10.1016/j.eco leng.2018.05.035.
Bulgariu, D.; Nacu, G.; Măluțan, T.; Bulgariu, L. (2016). Kinetic study of lead (II) removal from aqueous solution onto lignin-based materials. Cellulose Chemistry and Technology. 50(2), 339-347.
Bulgariu, L.; Malutan, T.; Bulgariu, D. (2013). Removal of Pb(II) ions from aqueous solution by adsorption on lignin obtained from rape wastes. Bulletin of the Polytechnic Institute of Iasi, Section Chemistry and Chemical Engineering. LIX (LXIII) (1), 75-86.
Cara, I.G.; Trincă, L.C.; Trofin, A.E.; Cazacu, A.; Țopa, D.; Peptu, C.A.; Jităreanu, G. (2015). Assessment of some straw-derived materials for reducing the leaching potential of Metribuzin residues in the soil. Applied Surface Science. 358, 586-594. https://doi.org/10.1016/j.apsusc.2015.08.141.
Chen, C.; Wang, J. (2008). Removal of Pb2+, Ag+, Cs+ and Sr2+ from aqueous solution by brewery’s waste biomass. Journal of Hazardous Materials. 151, 65–70. https://doi.org/10.1016/j.jhazm at.2007.05.046.
Chen, Q.; Hills, C.D.; Yuan, M.; Liu, H.; Tyrer, M.J. (2008). Characterization of carbonated tricalcium silicate and its sorption capacity for heavy metals: a micron-scale composite adsorbent of active silicate gel and calcite. Hazardous Materials. 153(1-2), 775-783. https://doi.org/10.1016/j.jhazmat.2007.09.023.
Dagnall, R.M.; West, T.S.; Young, P. (1965). Determination of lead with 4-(2-pyridylazo)-resorcinol-1spectrophotome try and solvent extraction, In Talanta, 12. Pergamon Press Ltd, 583 – 588.
Depci, T.; Kul, A.R.; Önal, Y. (2012). Competitive adsorption of lead and zinc from aqueous solution on activated carbon prepared from Van apple pulp: study in single- and multi-solute systems. Chemical Engineering Journal. 200-202, 224-236. https://doi. org/10.1016/j.cej.2012.06.077.
Erdem, M.; Ucar, S.; Karagöz, S.; Tay, T. (2013). Removal of Lead (II) Ions from Aqueous Solutions onto Activated Carbon Derived from Waste Biomass. The Scientific World Journal. 2013, 7. http://doi.org/10.1155/2013/146092.
Esmaili, A.; Nasseri, S.; Mahvi, A.H.; Atash-Dehghan, R. (2003). Adsorption of lead and zinc ions from aqueous solutions by volcanic ash soil(vas). Proceedings of the Eighth International Conference on En-vironmental Science and Technology. B, 193–199.
Fadillah, G.; Saleh, T.A.; Wahyuningsih, S. (2019). Enhanced electrochemical degradation of 4-Nitrophenol molecule s using novel Ti/TiO2-NiO electrodes. Journal of Molecular Liquids. 289, 111108. https://doi.org/10.1016/j.moll iq.2019.111108.
Gîlcă, I.-A.; Popa, V.I. (2013). Study on biocidal properties of some nanoparticles based on epoxy lignin. Cellulose Chemistry and Technology. 47 (3-4), 239-245.
Ge, Y.; Li, Z. (2018). Application of Lignin and Its Derivatives in Adsorption of Heavy Metal Ions in Water: A Review. ACS Sustainable Chemistry & Engineering. 6 (5), 7181-7192. https://doi.org/10.1021/acssuschemeng.8b01345.
Gong, L.; Wu, H.; Shan, X.; Li, Z. (2021). Facile fabrication of phosphorylated alkali lignin microparticles for efficient adsorption of antibiotics and heavy metal ions in water. Journal of Environmental Chemical Engineering. 9(6), 106574. https://doi.org/10.1016/ j.jece.2021.106574.
Guo, C.; Gemeinhart, R.A. (2008). Understanding the adsorption mechanism of chitosan onto poly(lactide-co-glycolide) particles. European Journal of Pharmaceutics and Biopharmaceutics. 70(2), 597-604. https://doi.org/10.1016/j.ejpb.2008.06.008.
Hanif, M.A.; Tauqeer, H.M.; Aslam, N.; Hanif, A.; Yaseen, M.; Khera, R.A. (2017). Correct Interpretation of sorption mechanism by Isothermal, Kinetic and Thermodynamic models. International Journal of Chemical and Biochemical Sciences. 12, 53-67, ISSN 2226-9614.
Janyasuthiwong, S.; Phiri, S.M.; Kijjanapanich, P.; Rene, E.R.; Esposito, G.; Lens, P.N.L. (2015). Copper, lead and zinc removal from metal-contaminated wastewater by adsorption onto agricultural wastes. Environmental Technology. 36(24), 3071-3083. https://doi.org/10.1080/ 09593330.2015.1053537.
Kurniawan, T.A.; Chan, G.Y.; Lo, W.H.; Babel S. (2006). Comparisons of low-cost adsorbents for treating wastewaters laden with heavy metals. Science of the Total Environment. 366(2-3), 409-426. https://doi. org/10.1016/j.scitotenv.2005.10.001.
Lin, X.; Shen, T.; Li, M.; Shaoyu, J.; Zhuang, W.; Li, M.; Xu, H.; Zhu, C.; Ying, H.; Ouyang, P. (2022). Synthesis, characterization, and utilization of poly-amino acid-functionalized lignin for efficient and selective removal of lead ion from aqueous solution. Journal of Cleaner Production. 347, 131219. https://doi.org/10.1016/j.jclepro.2022.131219.
Meng, J.; Tao, M.; Wang, L.; Liu, X.; Xu, J. (2018). Changes in heavy metal bioavailability and speciation from a Pb-Zn mining soil amended with biochars from co-pyrolysis of rice straw and swine manure. Science of The Total Environment. 633, 300-307. https://doi. org/10.1016/j.scitotenv.2018.03.199.
Nacu, G.; Negrilă, L.; Rosca, M.; Smaranda, C.; Bulgariu, L.; Gavrilescu, M. (2015). Efficient removal of Pb (II) ions from accidental polluted waters by adsorption onto thermal activated lignin. E-Health and Bioengineering Conference (EHB), November 19-21, Iaşi, Romania. https://doi.org/10.1109/EHB.2015.7391489.
Ponomarev, N.; Pastushok, O.; Repo, E.; Doshi, B.; Sillanpää, M. (2019). Lignin-Based Magnesium Hydroxide Nanocomposite. Synthesis and Application for the Removal of Potentially Toxic Metals from Aqueous Solution. ACS Applied Nano Materials. 2(9), 5492-5503. https://doi.org/10.10 21/acsanm.9b01083.
Rao, L.N.; Prabhakar, G.; Feroz, S. (2010). Adsorption of lead and zinc from aqueous solution using Ficus Benghalensis L. as adsorbent. International Journal of Engineering Studies. 2(2), 231-246. http://www.ripu blication.com/ijes.htm.
Ruthran, V.B.; Barman, P.; Kadam, R.; Kumar, A. (2021). Lignin-based adsorbent for effective removal of toxic heavy metals from wastewater. Emergent Materials. 34. https://doi.org/ 10.1007/s42247-021-00311-5.
Saleh, T.A. (2016). Nanocomposite of carbon nanotubes/silica nanoparticles and their use for adsorption of Pb(II): from surface properties to sorption mechanism. Desalination and Water Treatment. 57 (23). https://doi.org/ 10.1080/19443994.2015.1036784.
Saleh, T.A. (2020a). Characterization, determination and elimination technologies for sulfur from petroleum: Toward cleaner fuel and a safe environment. Trends in Environmental Analytical Chemistry. 25, e00080. https://doi.org/10.1016/j.teac.2020.e00080.
Saleh, T.A. (2020b). Trends in the sample preparation and analysis of nanomaterials as environmental contaminants. Trends in Environmental Analytical Chemistry. 28, e00101. https://doi.org/10.1016/j.teac.2020.e00101.
Saleh, T.A. (2020c). Nanomaterials: Classification, properties, and environmental toxicities, Environmental Technology & Innovation. 20, 101067. https://doi.org/10.1016/j.eti.2020.101067.
Saleh, T.A. (2021). Protocols for synthesis of nanomaterials, polymers, and green materials as adsorbents for water treatment technologies, Environmental Technology & Innovation. 24, 101821. https://doi.org/10.1016/j.eti.2021.101821.
Saleh, T.A. (2022). Experimental and analytical methods for testing inhibitors and fluids in water-based drilling environments. TrAC Trends in Analytical Chemistry. 149, 116543. https://doi.org/10.1016/j.trac.2022.116543.
Sani, H.A.; Ahmad, M.B.; Hussein, M.Z.; Ibrahim, N.A.; Musa, A.; Saleh, T.A. (2017). Nanocomposite of ZnO with montmorillonite for removal of lead and copper ions from aqueous solutions. Process Safety and Environmental Protection. 109, 97-105. https://doi. org/10.1016/j.psep.2017.03.024.
Sellaoui, L.; Mendoza-Castillo, D.I.; Reynel-Ávila, H.E.; Ávila-Camachod, B.A.; Díaz-Muñoz, L.L.; Houcine, G.; Bonilla-Petriciolet, A.; Abdelmottaleb, B.L. (2019). Understanding the adsorption of Pb2+, Hg2+ and Zn2+ from aqueous solution on a lignocellulosic biomass char using advanced statistical physics models and density functional theory simulations. Chemical Engineering Journal. 365, 305-316.
Song, M.; Wei, Y.; Cai, S.; Yu, L.; Zhong, Z.; Jin, B. (2018). Study on adsorption properties and mechanism of Pb2+ with different carbon based adsorbents. Science of The Total Environment. 618, 1416-1422. https://doi.org/10.1016/j.sci totenv.2017.09.268.
Tehrani, M.S.; Ghasemi, J.B.; Baharifard, M.T. (2012). Simultaneous spectrophotometric determination of zinc, cadmium and lead by xylenol orange using the partial least squares method after their preconcentration by 5,10,15,20-Tetrakis(4-Carboxylphenyl) porphyrin on Amberlite IRA-402 resin, Asian Journal of Chemistry. 24(7), 3078-3086.
Trakal, L.; Komárek, M.; Száková, J.; Zemanová, V.; Tlustoš, P. (2011). Biochar application to metal-contaminated soil: Evaluating of Cd, Cu, Pb and Zn sorption behavior using single- and multi-element sorption experiment, Plant, Soil and Environment. 57(8), 372-380. https:// doi.org/10.17221/155/2011-PSE.
Ungureanu, E.; Trofin, A.E.; Ariton, A.M.; Jităreanu, C.D.; Ungureanu, O.; Gîlcă, V.; Bors, S.I.; Popa, V.I. (2016). Applications of epoxidated lignins for bioprotection of lignocellulosic materials. Cellulose Chemistry and Technology. 50(1), 77-85.
Wang, B.; Sun, D.; Yuan, T.Q.; Song, G.; Sun, R.C. (2021). Recent Advances in Lignin Modification and Its Application in Wastewater Treatment. Lignin Utilization Strategies: From Processing to Applications: chapter 7, pages 143-173. https://doi.org/10.1021/bk-2021-1377.ch007.
Xu, X.; Cao, X.; Zhao, L. (2013). Comparison of Rice Husk- and Dairy Manure-Derived Biochars for Simultaneously Removing Heavy Metals from Aqueous Solutions: Role of Mineral Components in Biochars. Chemosphere. 92, 955-961. http://doi.o rg/10.1016/j.chemosphere.2013.03.009
Xue, C.; Zhu, L.; Lei, S.; Liu, M.; Hong, C.; Che, L.; Wang, J.; Qiu, Y. (2020). Lead competition alters the zinc adsorption mechanism on animal derived biochar. Science of the Total Environment. 713, 136395. https://doi. org/10.1016/j.scitotenv.2019.136395.
Academic Editor: Dr. Isabela Simion
Publisher Note: Regarding jurisdictional assertions in published maps and institutional affiliations ALSE maintain neutrality.
Eperjessy Diana Beatrice, Fortună Maria Emiliana, Trincă Lucia Carmen, Trofin Alina Elena, Ungureanu Elena