M.G. Azam, M.A. Hossain, J. Hossain, M.A. Hossain, M.O. Ali
ABSTRACT. The evaluation and computation of yield stability of a genotype over environments is a critical component of a certain breeding program. The present study was intended to screen 11 advance chickpea (Cicer arietinum L.) genotypes and one check for genotype × environment interaction (G × E) at six locations with varying micro and macro climatic conditions for yield correlated phenotypic characters. A number of 11 advanced genotypes of chickpea and one check variety were assessed for their adaptability at six different locations of Bangladesh. The randomized complete block design (RCBD) with three replications was chosen to experiment. The means were used to compute Additive Main Effects and Multiplicative Interaction (AMMI) analysis of variance, followed by regression analysis to measure × E. The regression analysis showed significant genotype × environment interaction for all the phenotypic characters. The mean values of days to flowering, days to maturity, plant height, number of pods per plant and seed yield were highly significant for linear, as well as non-linear components of G × E. Chickpea yield was significantly (p< 0.01) affected by genotypes, the environments and G × E interaction, indicating that the varieties and the test environments were diverse. G × E was further partitioned by principal component axes. The first two principal components cumulatively explained 86.59% of the total variation, of which 53.34% and 33.25% were contributed by IPCA1 and IPCA2, respectively. The AMMI stability value discriminated genotypes G2 (BCX 09010-9), G3 (BCX 09010-2) and G8 (BCX 01008-4) the stable genotypes. The investigated genotypes exhibited varying adaptability in different environments. Genotypes G3 (BCX 09010-9) and G9 (BCX 01008-3) were stable genotypes with high yield over a wide range of environments are promising candidate chickpea varieties.
Keywords: radicle length; radicle weight; organ extract; coleoptile length; coleoptile weight.
View full article (HTML)
Adaptibility Performances of Chickpea (Cicer Arietinum L.) Genotypes under Different Environments for Stability of Quantitative Traits
M.G. Azam1,*, M.A. Hossain2, J. Hossain1, M.A. Hossain1, M.O. Ali3
1Pulses Research Centre, BARI, Ishurdi, Pabna, Bangladesh
2Department of Genetics and Plant Breeding, BAU, Mymensingh, Bangladesh
3Pulses Research Sub-Station, BARI, Gazipur, Bangladesh
*E-mail: azam0r@yahoo.com
Received: Feb. 03, 2019. Revised: Feb. 24, 2020. Accepted: Mar. 09, 2020. Published online: June 5, 2020
ABSTRACT. The evaluation and computation of yield stability of a genotype over environments is a critical component of a certain breeding program. The present study was intended to screen 11 advance chickpea (Cicer arietinum L.) genotypes and one check for genotype × environment interaction (G × E) at six locations with varying micro and macro climatic conditions for yield correlated phenotypic characters. A number of 11 advanced genotypes of chickpea and one check variety were assessed for their adaptability at six different locations of Bangladesh. The randomized complete block design (RCBD) with three replications was chosen to experiment. The means were used to compute Additive Main Effects and Multiplicative Interaction (AMMI) analysis of variance, followed by regression analysis to measure × E. The regression analysis showed significant genotype × environment interaction for all the phenotypic characters. The mean values of days to flowering, days to maturity, plant height, number of pods per plant and seed yield were highly significant for linear, as well as non-linear components of G × E. Chickpea yield was significantly (p< 0.01) affected by genotypes, the environments and G × E interaction, indicating that the varieties and the test environments were diverse. G × E was further partitioned by principal component axes. The first two principal components cumulatively explained 86.59% of the total variation, of which 53.34% and 33.25% were contributed by IPCA1 and IPCA2, respectively. The AMMI stability value discriminated genotypes G2 (BCX 09010-9), G3 (BCX 09010-2) and G8 (BCX 01008-4) the stable genotypes. The investigated genotypes exhibited varying adaptability in different environments. Genotypes G3 (BCX 09010-9) and G9 (BCX 01008-3) were stable genotypes with high yield over a wide range of environments are promising candidate chickpea varieties.
Keywords: radicle length; radicle weight; organ extract; coleoptile length; coleoptile weight.
INTRODUCTION
Chickpea (Cicer arietinum L.) belongs to family Fabaceae. Chickpea contributes 20% of global food production (Broughton et al., 2003). In Bangladesh, it is grown on an area of 6.1 thousand ha with an annual production of 7.7 thousand tons and an average production of 1260 kg/ha (Anonymous, 2019). Chickpeas are not only an outstanding source of fiber, minerals and rich in protein but also used to decrease cholesterol and blood sugar levels. Chickpea contains fat in trace amounts. Chickpea is largely produced in rainfed areas of Bangladesh. The chickpea crop shows differential performance when grown under different environments, after the harvest of rice.
Environmental factors, such as soil moisture, sowing time, fertility, and temperature and day length have powerful influence during various stages of plant growth (Tilahun et al., 2015a).
The climate is changing day-by-day and this indicates that it is important to evaluate crop genotypes, at different environments, by assessment of performances.
Chickpea varieties, published so far, are adaptable to only favorable environments; therefore, most of them have narrow adaptability, as reflected by reduced gene expression of yield associated traits. Generally, in multi environmental evaluation of genotype, instability account less for the low potential of crops rather than low productivity. Thus, we can define stability as the repeatability of performance in different locations. An alteration in phenotypic characters of crops has been informed by many workers, due to soil physicochemical composition and environment interaction (Arunkumar et al., 2014; Shivani and Sreelakshmi 2014). Tolessa et al. (2013) define G × E interaction as variable phenotypic expression of genotypes in the response to environmental changes. This can be performed by the evaluation of genotypes in different environments.
All over the world, genotype × environment interaction has been investigated in different crops, before the final judgment of genotype as candidate variety. Shivani and Sreelakshmi (2014) reported significant variation for G × E interaction for grain yield in pigeon pea, Abo-Hegazy et al. (2013) in lentil.
Chickpea, also shows a high level of variability in performance with a change in climatic conditions (Bala et al., 1994).
Although Bakhsh et al. (1995) and Singh and Singh (2013) found some stable chickpea genotypes observed for grain. Keeping in mind the influence of G × E interaction it is compulsory to produce widely adapted elite varieties with stability in yield and yield associated traits under diverse agro-ecological conditions of Bangladesh. The assessment of stability and adaptability of the genotypes is an important component of any breeding program.
Various environmental factors affect plant growth at different stages of plant development (Bull et al., 1992; Oladosu et al., 2017). A stable genotype can be identified by considering the regression coefficient (bi) and deviation from regression (S2di) simultaneously.
A genotype having b<1.0 is considered to be more stable for low-performing environments, and a cultivar with b>1.0 has below-average stability, that is suitable for high-performing environments. A cultivar with b = 1.0 has average stability and is well or poorly adapted to all environments with high or low mean performance. Hence, a cultivar with b = 1.0 and S2d = 0.00 can be defined as a stable one (Eberhart and Russell, 1966). The exploitation of G × E interactions is important for the identification of stable varieties.
So, the present study was undertaken to assess the performance of different chickpea genotypes and their interaction with the environment at different locations along with stability parameters, for the identification of high-yielding and stable varieties with wider flexibility over environments.
MATERIAL AND METHODS
To screen advance chickpea genotypes for wide adaptability, six different locations (Ishurdi, Gazipur, Jessore, Barisal, Madaripur and Rajshahi) were selected for contrasting climatic conditions (Table 1). The seeds were sown during Rabi seasons and the experiment was laid out in a randomized complete block design with three replications. Therefore, 11 advance chickpea genotypes, subjected to stability analysis, were compared with one check (BARI Chola-9) for their stability across different environments (Table 2). Each entry was a sown in-unit plot of eight rows × 4 m long. The distance between the plants was 10 cm and that of the row was 50 cm. Seeds were sown in the rows carefully by hands at 3 cm depth and then covered by soils. Post sowing irrigation was given to ensure seed germination. Mulching was done and soil crusts were broken. Each of the entries was investigated from seedling to harvest and compared with the check.
Following the standard procedure, the data were recorded on yield traits, viz. days to 50% flowering, days to maturity, plant height (cm), number of pods/plant and seed yield (kg/ha). Yield and yield-related data were subjected to the Additive Main Effects and Multiplicative Interaction (AMMI) analysis of variance (ANOVA). ANOVA was used to partition genotype deviations from the grand mean, environment deviations from the grand mean, and GE deviations from the grand mean. The GEA-R software was used for ANOVA and stability analysis. The regression coefficient (bi) was measured according to Finlay and Wilkinson (1963) and Eberhart and Russell (1966) to determine the stability. According to Eberhart and Russell (1966), the regression coefficients approximating one coupled with (S2di) of zero indicate average stability.
Table 1
Environments used in the study and their main characteristics
Environments |
Rainfall (mm) |
Temp.
|
Longitude (E) |
Latitude (N) |
Altitude (m) |
Soil type |
Rajshahi (E1) |
22 |
6.12-28.8oC |
88°42ʹ |
24°480ʹ |
40 |
Clay loam |
Gazipur (E2) |
00 |
12.16 -31.40oC |
90°25ʹ |
24°03ʹ |
8.4 |
Clay loam |
Ishurdi (E3) |
00 |
10.33-19.35oC |
89°12ʹ |
24°071ʹ |
19 |
Clay loam |
Madaripur (E4) |
00 |
6.02-32.8oC |
90°19ʹ |
23°239ʹ |
9 |
Silty loam and silty clay loam |
Barishal (E5) |
00 |
10.32-29.02oC |
90°32ʹ |
22°816ʹ |
7 |
Silty clay |
Jashore (E6) |
00 |
8.79-22.07oC |
89°18ʹ |
23°177ʹ |
14 |
Clay loam |
Table 2
Name, origin and code of chickpea genotypes tested in six environments
Genotype names |
Genotype code |
Origen |
Genotype code |
Genotype names |
Origen |
ICCV 93954 |
G1 |
ICARDA |
G9 |
BCX 01008-3 |
BARI |
BCX 09010-9 |
G2 |
BARI |
G10 |
ICCV 07105 |
ICARDA |
BCX 09010-2 |
G3 |
BARI |
G11 |
ICCV 060157-3 |
ICARDA |
ICCV 07102 |
G4 |
ICARDA |
G12 |
BARI Chola-9 |
BARI |
ICCV 12115 |
G5 |
ICARDA |
|
|
|
ICCV 12110 |
G6 |
ICARDA |
|
|
|
BCX 01008-8 |
G7 |
BARI |
|
|
|
BCX 01008-4 |
G8 |
BARI |
|
|
|
RESULTS AND DISCUSSION
In Fig. 1, the horizontal blue line showed the interaction score of zero and the vertical blue line indicated the grand mean yield. X-coordinate means the principal effects and the y-coordinate points out the effects of the interaction (IPCA1). These were considered as high yielding genotypes and environments. Values closer to the origin of the axis (IPCA1) provide a smaller contribution to the interaction than those that are further away. Accordingly, the AMMI1graph shows that G1 and G2 genotypes stood out with the lowest IPCA1 scores (Fig. 1). This indicates that these were the least involved with the interaction and are therefore the most stable. However, only the yield of G2 genotypes was above-average. On the other hand, the genotypes G11, G10 and G6 were the most unstable, G6 with the highest average yield. Some of the environments stood out with a small contribution to the interaction (E2), with an intermediate contribution (E1 and E5), and with a high contribution (E3, E4 and E6) (Fig. 1). The most ideal genotype should combine high yield and stable performance across a range of production environments. Among the six high yielding genotypes G2, G3, G4, G6, G8 and G12; G2 and G3 genotypes can be best evaluated based on stability and grain yield with combined low absolute PC1 score and high yield (Fig. 1).
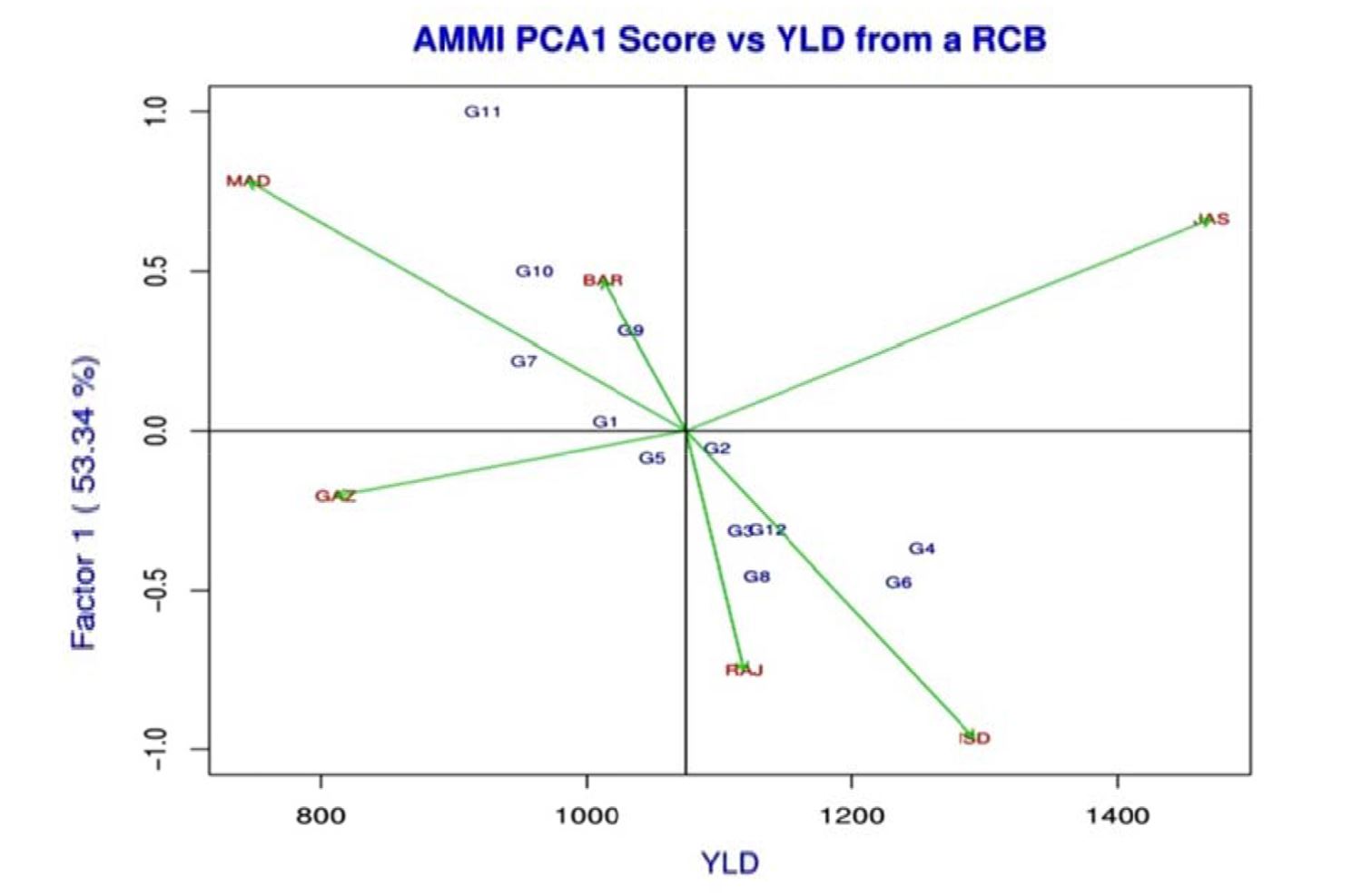
Figure 1 – Plot of genotype and environment IPCA 1 scores versus grand means of yield. G1 – G12 with blue color represent genotypes, while environments are represented by red color. The details of the genotypes and environments are presented in Tables 1 and 2.
AMMI 2 biplot presents the pattern of the first two IPCA of the interaction effects and aids in the visual interpretation of the G × E interaction patterns and identifies genotypes or environments that show small and large interaction effects. In AMMI 2 biplot, environments fell into three sections (Fig. 2). Among the environments, GAZ had very short-spokes and BAR, JAS & MAD had short spokes. They do not exert strong interaction, but the environments RAJ and ISD had long spokes and hence show the most influencing environments. In AMMI 2 biplot, the genotypes, G9, G4, G6, G1 and G11 are the best or poorest genotypes in some or all environments, because they are farthest from the origin, whereas the best genotype is G11 with respect to the best-enhancing environment JAS and MAD and the poor genotype is G9 due to its value below-average value. On the other hand, the genotypes G2, G5 and G12 were close to the origin and therefore were less/non-sensitive to environmental interaction. The entries G2 and G12 were the highest yielding and stable due to very close to the origin. AMMI stability value (ASV) discriminated genotypes G2 (BCX 09010-9), G3 (BCX 09010-2) and G8 (BCX 01008-4) the stable accessions. Similar results were reported by Crossa (1990), Lule et al. (2014) and Gebre (2014).
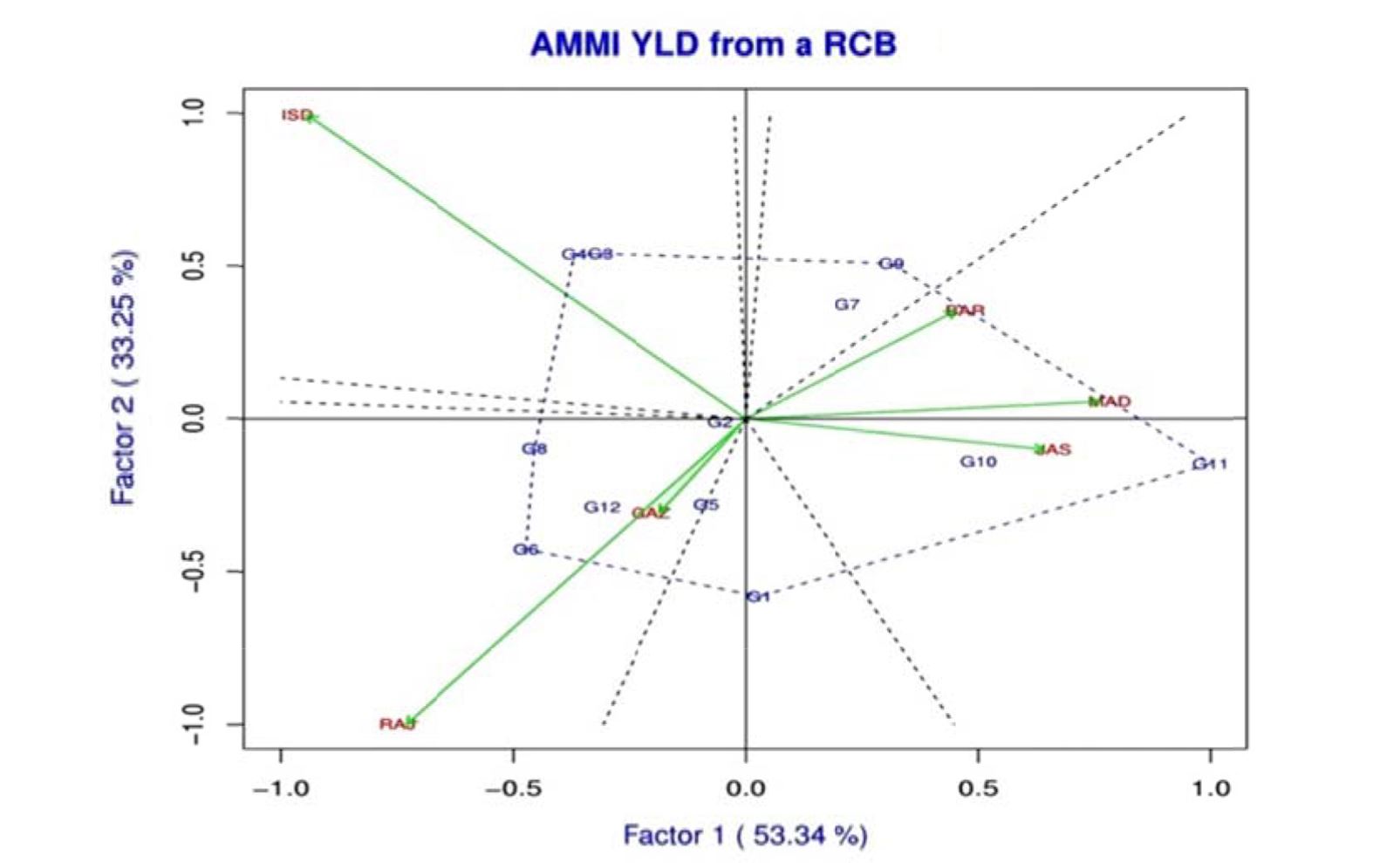
Figure 2 – Plot of IPCA1 (factor 1) versus IPCA2 (factor 1) scores. G1 – G12 with blue color represent genotypes, while environments are represented by red color. The details of the genotypes and environments are presented in Tables 1 and 2.
Results of analysis of variance for stability analysis for seed yield and its components (Table 3) revealed that mean squares due to genotype were significant for all the traits indicated significant differences among them. Mean squares due to environment (linear) were significant for all the traits that mean significant differences among the environments. Mean squares, due to genotypes × environments (linear), were significant for all yield-related traits and seed yield indicated that the varieties differed genetically for their regression on the environmental index and hence the performance is predictable for all traits and seed yield. These findings are in general agreement with the findings reported by (Rao, 2011; Shivani and Sreelakshmi 2015; Tilahun et al., 2015a; Tilahun et al., 2015b; Yadav et al., 2010; Yadav et al., 2014; Rao and Rao 2004).
Table 3
ANOVA for AMMI analysis of genotype x environment interaction of chickpea genotypes
Source of variation |
DF |
Mean sum of squares (MS) |
||||
Days to 50% flowering |
Days to maturity |
Plant height |
Pods/ plant |
Seed yield (kg/ha) |
||
Environments (E) |
5 |
2707.42*** |
3151.98*** |
1081.82*** |
8268.72*** |
3055981.72*** |
Genotypes (G) |
11 |
70.84*** |
166.77*** |
511.15*** |
612.68*** |
447386.30** |
Interaction (G×E) |
55 |
39.28*** |
108.07*** |
79.00*** |
260.68*** |
301118.93 |
IPCA1 |
15 |
102.25*** |
284.33*** |
151.21*** |
478.80*** |
868245.44* |
IPCA2 |
13 |
23.09*** |
77.01*** |
97.02*** |
341.32*** |
188854.09 |
IPCA3 |
11 |
14.44*** |
35.94*** |
45.67*** |
192.91*** |
59419.54 |
Residuals |
144 |
1.25 |
2.02 |
0.92 |
1.37 |
247448.79 |
Error |
132 |
– |
– |
– |
– |
– |
Significance codes: ***0.001, **0.01 and *0.01 |
According to Eberhart and Russell (1966), a stable genotype is one with a high mean, a regression coefficient of unity (bi = 1), and a minimum deviation from the regression coefficient (S²di) = 0 or close to these values of non-significant deviation. Thus, this analysis allows the identification of stable genotype for trait across environments and of genotypes that are the most responsive to a favorable or unfavorable environment. The estimates of stability parameters in respect of six characters that had a direct influence on genotype performance are presented in Table 4.
Results of stability parameters revealed that for days to 50% flowering varieties G9, G11, G5, G12 and G4 exhibited the days to 50% flowering early to the average and regression significantly differed from zero. For days to maturity, varieties G5, G9, G3, G11 and G7 exhibited earliness, compared to the average and regression significantly differed from zero. For plant height varieties G12, G6, G11, G10 and G4 showed short plant height to the average and regression significantly differed from zero. Little plants are more desirable of plant breeders due to less attack by insects and diseases. For pods/plant, varieties G5, G2, G1, G4 and G8 exhibited the number of pods/plant to the average and non-significant regression and deviation from regression. In the present study, genotypes G3, G9 and G8 had higher mean yield, unit regression coefficient (bi=1) and non-significant S²di (Table 4). Thus they were found to be stable, high yielding genotypes that can be adapted to all the environments.
The grand mean of seed yield over all locations was 1103 kg ha-1. G11, G12, G2, G8 and G7 produced above-average yield, but were not stable, either due to the significant regression coefficient or significant deviation from regression.
The remaining genotypes, viz. G5, G4, G1, G6, and G10 had a below-average yield. Stability in the seed yield had been previously reported by Saleem et al. (2002), Swamy and Reddy (2004), Sarkar and Kundagrami (2017) in mungbean, Bakhsh et al. (2006) and Prakash (2006) in chickpea, Thakare et al. (2016) in rajmash, Karimizadeh et al. (2012) in lentil and Temesgen et al. (2015) in faba bean.
The level of response of different genotypes toward stability parameters was different. Genotypes having stable performance, under different environmental conditions, may be released as a commercial variety or used in a breeding program for the evolution of high-yielding and stable chickpea varieties.
Performance of different chickpea genotypes, under varying environmental conditions, indicated that G3 (BCX 09010-9) and G9 (BCX 01008-3) were the most stable genotype, which showed more seed yield, compared with the grand mean. So, it was concluded that genotypes having a regression coefficient equal to or close to unity level, with above-average value were stable, over a wide range of environmental conditions (Eberhartand Russell, 1966; Finaly and Wilkinson, 1963). Genotypes above unity level were high yielding only in favorable environments, while genotypes below unity expressed unpredictable performance.
CONCLUSIONS
The mean values of days to flowering, days to maturity, plant height, pods per plant and seed yield were highly significant for linear, as well as non-linear components of G × E.
The investigated genotypes exhibited varying adaptability at different environments. Genotypes G3 (BCX 09010-2) and G9 (BCX 01008-3) were stable genotypes with high yield over a wide range of environments that are promising chickpea genotypes.
The AMMI stability analysis genotypes G2 and G12 were the highest yielding and stable, due to very close to the origin. AMMI stability value (ASV) discriminated genotypes G2 (BCX 09010-9), G3 (BCX 09010-2) and G8 (BCX 01008-4) the stable accessions. Therefore, this genotype is suitable for cultivation in these agro-ecological zones and could be utilized as a good breeding material in developing chickpea varieties with high adaptation.
REFERENCES
Abo-Hegazy, S.R.E., Selim, T. & Ashrie, A.A.M. (2013). Genotype x environment interaction and stability analysis for yield and its components in lentil. J. Plant Breed. Crop Sci., 5(5): 85-90, DOI: 10.5897/JPBCS 12.066
Anonymous (2019). Agriculture Information Centre. Ministry of Agriculture, GOB. Bangladesh.
Arunkumar, B., Muniswamy, S. & Dharmaraj, P.S. (2014). Interpretation of genotype environment interaction and stability analysis for grain yield of pigeon pea (Cajanus cajan L.).J.Appl.Nat.Sci., 6(2): 744-747, DOI: 10.31018/jans. v6i2.529
Bakhsh, A., Ghafoor, A., Zubair, M. & Iqbal, S.M. (1995). Genotype environment interaction for grain yield in lentil. Pak.J.Agric.Res., 12: 102-105.
Bakhsh, A., Arshad, M. & Haqqani, A.M. (2006). Effect of genotype x environment interaction on relationship between grain yield and its components in chickpea (Cicer arietinum L.). Pak.J.Bot., 38: 683-690.
Bala, H., Satija, D. & Gupta, V.P. (1994). Variation and association among various protein quality traits in chickpea. Ind.J.Genet. Plant. Br., 54: 105-109.
Broughton, W.J., Hernández, G., Blair, M., Beebe, S., Gepts, P. & Vanderleyden, J. (2003). Beans (Phaseolus spp.) model food legumes. Plant Soil, 252: 55-128.
Bull, J.K., Cooper, M., Delacy, I.H., Basford, K.E. & Woodruff, D.R. (1992). Utility of repeated checks for hierarchical classification of data from plant breeding trials. Field Crops Res., 30(1-2): 79-95, DOI: 10.1016/0378-4290(92)90058-H
Crossa, J. (1990). Statistical analyses of multi-location trials. Adv.Agron., 44: 55-85, DOI: 10.1016/S0065-2113 (08)60818-4
Eberhart, S.A. & Russell, W.A. (1966). Stability parameters for comparing varieties. Crop Sci., 6(1): 36-40, DOI: 10.2135/cropsci1966.0011183 X000600010011x
Finaly, K.W. & Wilkinson, G.N. (1963). The analysis of adaptation in a plant- breeding program. Aus.J.Agric.Res., 14(6): 742-756, DOI: 10.1071/AR9 630742
Karimizadeh, R., Mohammadi, M., Sabaghnia, N. & Shefazadeh, M.K. (2012). Using different aspects of stability concepts for interpreting genotype by environment interaction of some lentil genotypes. Aust.J. Crop Sci., 6(6): 1017-1023.
Lule, D., Fetene, M., de Villiers, S. & Tesfaye, K. (2014). Additive main effects and multiplicative interactions (AMMI) and genotype by environment interaction (GGE) biplot analyses aid selection of high yielding and adapted finger millet varieties. J.Appl.Biosci., 76: 6291-6303, DOI: 10.4314/jab.v76i1.1
Oladosu, Y., Rafii, M.Y., Abdullah, N., Magaji, U., Miah, G., Hussin, G. & Ramli, A. (2017). Genotype x Environment interaction and stability analysis of yield and yield components of established and mutant rice genotypes tested in multiple locations in Malaysia. ActaAgric.Scand.B, 67: 590-606, DOI: 10.1080/09064710.2017.1321 138
Prakash, V. (2006). Stability analysis for grain yield and contributing traits in chickpea (Cicer arietinum L.). Indian J.Genet., 66(3): 239-240.
Rao, M. & Rao, Y. (2004). Stability analysis in chickpea (Cicer arietinum L.). Legume Res., 27(4): 235-242.
Rao, P.J.M. (2011). Stability analysis for grain yield and yield components in chickpea (Cicer arietinum L.). Elect.J.Plant Breed., 2(1): 47-49.
Saleem, M., Sadiq, M.S., Sarwar, G. & Abbas, G. (2002). Performance of elite mungbean (Vigna radiata (L) Wilczek) germplasm in multilocational experiments. Biologia, 48: 9-13.
Sarkar, M. & Kundagrami, S. (2017). Germplasm x environment interaction and stability for seed yield components and maturity duration in mungbean. Int.J.Curr. Res., 9(2): 45993-45998.
Shivani, D. & Sreelakshmi, Ch. (2014). Genotype environment interaction and stability analysis in rabi sorghum. J. Global Biosci., 3(2): 626-632.
Shivani D. & Sreelakshmi, Ch. (2015). Stability analysis in chickpea (Cicer arietinum L.) J. Global Biosci., 4(7): 2822-2827.
Singh, A.K. & Singh, A.P. (2013). Study of genetic variability and interaction of some quantitative traits in chickpea (Cicer arietinum L). Technofame, 2(1): 87-94.
Swamy, A.A. & Reddy, G.L.K. (2004). Stability analysis of yield in mungbean (Vigna radiata L. Wilczek). Legume Res., 27(2): 107-110.
Temesgen, T., Keneni, G., Sefera, T. & Jarso, M. (2015). Yield stability and relationships among stability parameters in faba bean (Vicia faba L.) genotypes. Crop J., 3(3): 258-268, DOI: 10.1016/j.cj.2015.03.004
Thakare, P.A., Lad, D.B., Dalavi, N.D. & Bade, B.A. (2016). Stability analysis for seed yield and its components in rajmash (Phaseolus vulgaris L.) genotypes to different environments. Int.J. Pure App.Biosci., 4: 160-167, DOI: 10.18782/2320-7051.2396
Tilahun, G., Mekbib, F., Fikre, A. & Eshete, M. (2015a). Genotype x environment interaction and stability analysis for yield and yield related traits of Kabuli-type chickpea (Cicer arietinum L.) in Ethiopia. Afr.J.Biotechnol., 14(18): 1564-1575, DOI: 10.5897/AJB2014.14320
Tilahun, G., Mekbib, F., Fikre, A. & Eshete, M. (2015b). Genotype x environment interaction and stability analysis for yield and yield related traits of desi-type chickpea (Cicer arietinum L.) in Ethiopia. Curr.Res.Agric.Sci., 2(3): 100-113, DOI: 10.18488/journal.68/2015.2.3/ 68.3.100.113
Tolessa, T.T., Keneni, G., Sefera, T., Jarso, M. & Bekele, Y. (2013). Genotype x environment interaction and performance stability for grain yield and Field pea (Pisum sativum L.) genotypes. Int J. Plant Breed., 7(2): 116-123.
Gebre, W. (2014). Evaluation of pearl millet (Pennisetum glaucum L.) genotypes for yield and yield stability in South Omo and West Hararghe. JBAH, 4(8): 99-121
Yadav, S.S., Verma, A.K., Rizvi, A.H., Singh, D., Kumar, J. & Andrews, M. (2010). Impact of genotype × environment interactions on the relative performance of diverse groups of chickpea (Cicer arietinum L.) cultivars. Arch.Agron. Soil Sci., 56(1): 49-64, DOI: 10.1080/036503 40902922688
Yadav, Asha, Yadav, I.S. & Yadav, C.K. (2014). Stability analysis of yield and related traits in chickpea (Cicer arietinum L.). Legume Res., 37(6): 641-645, DOI: 10.5958/0976-0571. 2014.00689.4