Vasile Răzvan Filimon, Roxana Mihaela Filimon, Ancuța Nechita, Florin Dumitru Bora, Liliana Rotaru, Valeriu V. Cotea
ABSTRACT. Nowadays, consumers go towards products that provide food security and nutritional richness, consumption of highly alcoholic beverages not complying with these health-related requirements. This study aimed to obtain low-alcohol wines by performing sequential alcoholic fermentation of grape must (Muscat Ottonel). Thus, 11 commercial yeast strains (10 Saccharomyces cerevisiae and 1 Torulaspora delbrueckii) were preliminarily tested in anaerobic and semi-anaerobic conditions. Based on laboratory tests, grape must fermentation was sequentially initiated in semi-anaerobic conditions (loose cotton plugs; intermittent homogenization), with the T. delbrueckii strain (106 CFU/mL) and the addition of preliminarily selected S. cerevisiae strains (104 CFU/mL), to the consumption of 50% of the sugars in the must, to complete the fermentation. By applying this technology, dry wines were obtained with an alcohol content lower by up to 1.10% vol., but with a lower concentration of volatile terpenes, due to additional must oxidation. Also, semi-anaerobiosis enhanced glycerol synthesis by yeasts (< 35%), with a positive impact on the sensory characteristics of the wine.
Keywords: alcoholic fermentation; glycerol; low-alcohol wine; non-Saccharomyces yeast; Torulaspora delbrueckii.
Cite
ALSE and ACS Style
Filimon, V.R.; Filimon, R.M.; Nechita, A.; Bora, F.D.; Rotaru, L.; Cotea, V.V. The production of low-alcohol wines by sequential semi-anaerobic must fermentation. Journal of Applied Life Sciences and Environment 2022, 55 (4), 407-418.
https://doi.org/10.46909/alse-554073
AMA Style
Filimon VR, Filimon RM, Nechita A, Bora FD, Rotaru L, Cotea VV. The production of low-alcohol wines by sequential semi-anaerobic must fermentation. Journal of Applied Life Sciences and Environment. 2022; 55 (4): 407-418.
https://doi.org/10.46909/alse-554073
Chicago/Turabian Style
Filimon, Vasile Răzvan, Roxana Mihaela Filimon, Ancuța Nechita, Florin Dumitru Bora, Liliana Rotaru, and Valeriu V. Cotea. 2022. “The production of low-alcohol wines by sequential semi-anaerobic must fermentation” Journal of Applied Life Sciences and Environment 55, no. 4: 407-418.
https://doi.org/10.46909/alse-554073
View full article (HTML)
The Production of Low-Alcohol Wines by Sequential Semi-Anaerobic Must Fermentation
Vasile Răzvan FILIMON1*, Roxana Mihaela FILIMON1, Ancuța NECHITA1, Florin Dumitru BORA2, Liliana ROTARU3 and Valeriu V. COTEA3
1Research Development Station for Viticulture and Winemaking, 48, Mihail Sadoveanu Alley, 700489, Iasi, Romania
2University of Agricultural Sciences and Veterinary Medicine, 3-5, Mănăștur Street, 400372, Cluj-Napoca, Romania
3Iasi University of Life Sciences, Faculty of Horticulture, 3, Mihail Sadoveanu Alley, 700490, Iasi, Romania
*Correspondence: razvan_f80@yahoo.com
Received: Feb. 22, 2023. Revised: Mar. 22, 2023. Accepted: Apr. 03, 2023. Published online: Apr. 11, 2023
ABSTRACT. Nowadays, consumers go towards products that provide food security and nutritional richness, consumption of highly alcoholic beverages not complying with these health-related requirements. This study aimed to obtain low-alcohol wines by performing sequential alcoholic fermentation of grape must (Muscat Ottonel). Thus, 11 commercial yeast strains (10 Saccharomyces cerevisiae and 1 Torulaspora delbrueckii) were preliminarily tested in anaerobic and semi-anaerobic conditions. Based on laboratory tests, grape must fermentation was sequentially initiated in semi-anaerobic conditions (loose cotton plugs; intermittent homogenization), with the T. delbrueckii strain (106 CFU/mL) and the addition of preliminarily selected S. cerevisiae strains (104 CFU/mL), to the consumption of 50% of the sugars in the must, to complete the fermentation. By applying this technology, dry wines were obtained with an alcohol content lower by up to 1.10% vol., but with a lower concentration of volatile terpenes, due to additional must oxidation. Also, semi-anaerobiosis enhanced glycerol synthesis by yeasts (< 35%), with a positive impact on the sensory characteristics of the wine.
Keywords: alcoholic fermentation; glycerol; low-alcohol wine; non-Saccharomyces yeast; Torulaspora delbrueckii.
INTRODUCTION
Over time, the alcoholic concentration of wines has evolved progressively, a trend that was initially attributed to climate change, i.e. a gradual increase in temperatures, which led to high accumulation of carbohydrates in grapes (Canonico et al., 2019; Garcia et al., 2020). This trend was later correlated with the improvement of agrotechnical practices, selection of genotypes with high capacity to accumulate sugars in berries or the use of osmotolerant, highly alcoholic yeasts (Filimon et al., 2020).
Microorganisms that produce alcoholic fermentation constitute a very large group of organisms (mainly yeasts and bacteria), different in nature, form and biological activity. Yeasts are responsible for the alcoholic fermentation of grape must, converting sugars into alcohol, carbon dioxide and various concentrations of other by-products (Romano et al., 2020). Nowadays, the use of selected yeasts for the initiation and control of alcoholic fermentation has become a current practice, even for producers with limited material possibilities.
Saccharomyces cerevisiae is generally recognized as the main agent involved in the alcoholic fermentation of grape must (Nadai et al., 2019). However, strains belonging to other yeast genera, including Hanseniaspora/ Kloeckera, Pichia, Candida, Lachancea, Torulaspora or Metschnikowia, predominate during the first stages of alcoholic fermentation (< 3–4% vol.) (Fleet and Heard, 1993). Among these, Torulaspora delbrueckii is the most studied and most frequently isolated and tested yeast for use in winemaking (Ramirez and Velázquez, 2018). This is because T. delbrueckii can be used to optimize some chemical parameters of wine, such as obtaining low volatile acidity, reducing the alcoholic concentration of wines, producing a higher amount of glycerol, releasing mannoproteins and polysaccharides, forming higher amounts of aromatic compounds that enrich the aromatic profile of the wine (fruity aromas – esters, lactones, thiols and terpenes) and reducing the concentrations of undesirable compounds (Mehlomakulu et al., 2021).
Depending on the concentration of oxygen in the environment, the metabolic degradation of carbohydrates can follow two paths: that of oxidation (aerobic), with the formation of caloric energy, CO2 and H2O, and that of alcoholic fermentation (anaerobic), with the formation of alcohol, CO2, caloric energy and various secondary compounds. The aerobic metabolism of carbohydrates is associated with the respiration process (‘Pasteur’ effect), but some yeasts may produce ethanol even in aerobic conditions at high exogen glucose concentrations (‘Crabtree’ effect) (Gonzalez et al., 2013). Although yeasts can utilize sugars either through fermentation or respiration, aeration of culture media induces an increase in the amount of biomass and a decrease in alcohol production (Meiser and Vazquez, 2020). When the dissolved oxygen concentration increases, yeast switch from a fully fermentative metabolism to a mixed respiro-fermentative mechanism, ethanol production remaining the main metabolic direction (Aceituno et al., 2012). Moreover, Gonzalez et al. (2013) proposed the use of respiratory metabolism of non-Saccharomyces yeasts as a tool for reducing the alcohol content in wine. Although non-Saccharomyces yeasts are generally characterized by low fermentation vigour, from a physiological point of view T. delbrueckii is the closest species to S. cerevisiae (Mehlomakulu et al., 2021). For these reasons, T. delbrueckii was the first non-Saccharomyces yeast proposed for industrial grape must fermentation (Castelli, 1955).
Considering all these aspects, the purpose of this study was to obtain low-alcohol wines by manipulating the fermentative metabolism of some laboratory-selected commercial yeast strains, and to identify the conditions that induced the modification in the sugar/ethanol conversion rate, as an alternative approach to avoiding excess ethanol production during winemaking.
MATERIALS AND METHODS
Ten commercial Saccharomyces sp. yeast strains and a non-Saccharomyces strain, Torulaspora delbrueckii (Table 1), were tested in monoculture, in anaerobic and semi-anaerobic conditions. To obtain the inoculum, 0.1 g of each commercial yeast was rehydrated in water (20 min, 30°C), pre-cultured in 5 mL of liquid YPD medium (10 g/L yeast extract, 20 g/L peptone, 20 g/L dextrose; autoclaved 20 min at 121°C) and incubated for 48 h at 30 °C (Sadoudi et al., 2012).
Two hundred millilitres of sterile (10 min, 100°C) Muscat Ottonel must (171 g/L sugars, total acidity 3.82 g/L as tartaric acid, pH 3.52, 203 mg/L assimilable nitrogen), was separately inoculated with each yeast strain, at a cell density of 106 CFU (colony-forming units)/mL, and incubated at 20± 2°C (Biobase, Firlabo) for alcoholic fermentation. The Erlenmeyer flasks for anaerobic fermentation (500 cm3) were closed with a two-chamber unidirectional bubbler glass airlock, while the flasks for semi-anaerobic fermentation were closed with loose cotton plugs and homogenized for 20 s, once a day at sampling.
Yeast characteristics during the fermentation process (fermenting power, fermentation kinetics, growth in liquid medium) were assessed according to Resolution OIV-OENO 370-2012 (OIV, 2012). Cell growth was determined by measuring the absorbance at 600 nm and by weighing the cell biomass (ATX 224 analytical balance, Shimadzu, Japan) after centrifugation (7500 rpm, 10 min).
Based on laboratory experiments, non-sterile clarified Muscat Ottonel must (186 g/L sugars, total acidity 4.61 g/L as tartaric acid, pH 3.46, 166 mg/L assimilable nitrogen) was sequentially fermented at micropilot level (in 25 L glass demijohns), in semi-anaerobic conditions (loose cotton plugs), by initial inoculation with T. delbrueckii (106 CFU/mL), followed by the addition of the preliminarily selected S. cerevisiae strain inoculum (104 CFU/mL) to the consumption of 50% of the sugars in must. The Muscat Ottonel must, fermented in anaerobic conditions (fermentation airlocker) by inoculation with S. cerevisiae (106 CFU/mL) in monoculture, represented the control sample. Prior to this, active cells were pre-cultured for 48 h at 28°C, in grape must diluted 50% with distilled water, in order to shorten the lag phase of the non-Saccharomyces yeast. Also, the S. cerevisiae starter cells were acclimated for 1 h in 1 : 1 water : must medium before inoculation.
In order to ensure the antioxidant and antimicrobial protection of the wines, 100 mg/L of SO2 was added after racking wine off the lees. The main physicochemical parameters of grape musts and wines, including total polyphenolic content (TPC), were analysed according to the OIV Compendium of International Methods of Wine and Must Analysis (OIV, 2019). Glycerol concentrations were determined by enzymatic kit no. 12812 (BioSystems, Spain). Free volatile terpenes (FVT) and precursors (PVT) were evaluated using the method proposed by Dimitriadis and Williams (1984) (µg/L as linalool equivalent). Wine colour components (colour intensity, colour hue and the proportions of red, yellow and blue) were analysed according to Glories (1984), the absorbance of the samples being determined at 420, 520 and 620 nm. All spectral determinations were performed using a Specord 200 plus double-beam UV-vis spectrophotometer (Analytik Jena, Germany).
Sensory analysis of the wine was carried out by a panel of 13 initiated and expert tasters, scoring from 1 (poorest) to 10 (best) each of the 19 characteristics analysed.
Data are reported as the mean of a minimum of two replicates, with standard deviation (±).
Analysis of variance (ANOVA test) (Microsoft® Excel, Data Analysis) was initiated to investigate significant differences between data. p-values < 0.05 were considered to be significant (●).
RESULTS AND DISCUSSION
The preliminary tests performed with the 11 commercial yeasts tested in monoculture provided important information on their growth and development. Fermenting power (FP) in anaerobic conditions (20 ℃), determined by gravimetric analysis as the daily difference (Δ) in flask weight (FP = 2.5·Δ), was high, varying between 60.00 (T8) and 63.61 (T7) (Figure 1).
Table 1
Commercial yeast strains used in the experiments
Strain code | Product name | Producer | Yeast species |
T1 | Fermactive CB | Sodinal, France | Saccharomyces cerevisiae var. bayanus |
T2 | Fermactive Thiols | Sodinal, France | S. cerevisiae |
T3 | Zymasil Bayanus | AEB, France | S. bayanus (oviformis) |
T4 | SP39 | Sofralab, France |
S. cerevisiae galactose + S. cerevisiae |
T5 | Zymaflore X5 | Laffort, France | S. cerevisiae |
T6 | Zymaflore X16 | Laffort, France | S. cerevisiae |
T7 | Viniferm | Agrovin, Spain | S. cerevisiae |
T8 | Vinoferm Basic Cerevisiae | Essedielle, Italy | S. cerevisiae |
T9 | Vinoferm Basic Bayanus | Essedielle, Italy | S. bayanus |
T10 | Vinoferm Aroma | Essedielle, Italy | S. cerevisiae |
T11 | Zymaflore Alpha | Laffort, France | Torulaspora delbrueckii |
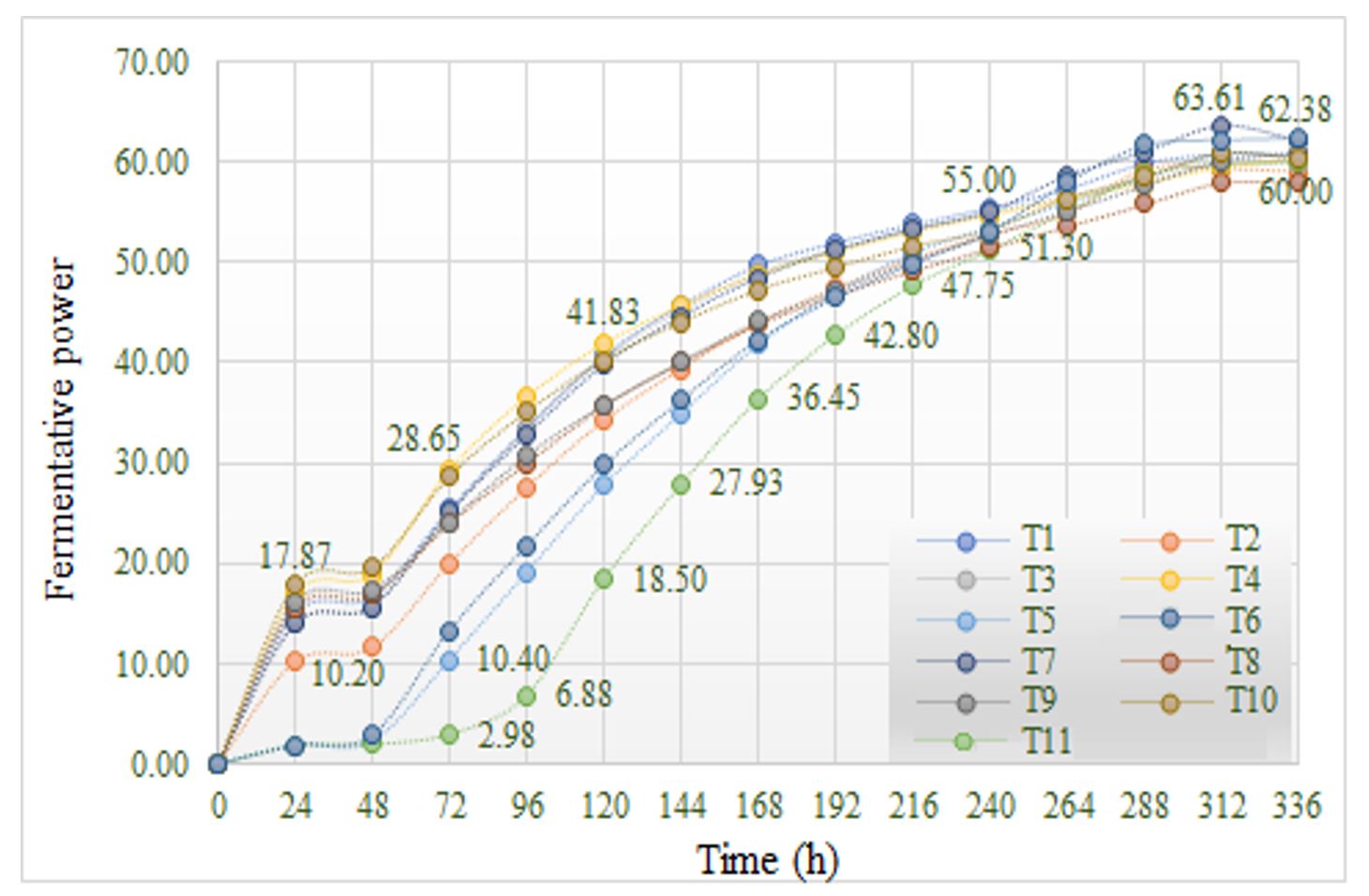
Figure 1 – Fermentative power of tested yeast strains in anaerobic conditions
Note: T1–T11 represent yeast codes as shown in Table 1.
After 14 days of anaerobic fermentation a different evolution of the FP was observed, in relation to sugar metabolization, ethanol synthesis and CO2 release. Strains T4 (SP39, S. cerevisiae) and T7 (Viniferm, S. cerevisiae) showed high values of FP throughout the fermentation (Figure 1).
In the case of strain T11 (T. delbrueckii), although it showed average final values of FP, in the first 3 days, the values for this parameter were the lowest compared to Saccharomyces yeast strains. Torulaspora cells have a spherical shape, smaller than those of S. cerevisiae, but its life cycle has not yet been fully elucidated. The delay in ethanol synthesis was partially explained by Alves-Araujo et al. (2007). Thus, unlike S. cerevisiae, that switches to a respiro-fermentative metabolism as the amount of oxygen decreases, T. delbrueckii was still able to sustain full respiration under low-oxygen conditions.
Similar to anaerobic fermentation, in semi-anaerobic conditions (limited gas circulation), all yeasts managed to consume over 99% of the sugars in the must, with residual sugar concentrations between 0.32 and 1.60 g/L (data not shown).
During the semi-anaerobic fermentation, the daily values of the cell density (OD 600 nm) increased gradually. The highest values were registered for strain T4 (SP39) (14.00 absorbance units), much higher than for the non-Saccharomyces strain (T11, T. delbrueckii), which showed the lowest values after 14 days of semi-anaerobic fermentation (10.24) (Figure 2).
A massive increase in the optical density at 600 nm was observed in the first 7 days of the semi-anaerobic process (exponential growth phase), after a lag phase of 12–48 h, depending on the strain. The T. delbrueckii strain (T11) showed different kinetics than did Saccharomyces strains, respectively a longer adaptation phase to environmental conditions (3 days) and a shorter exponential growth phase (approx. 4 days), the plateau phase being reached at the same time as in the Saccharomyces strains.
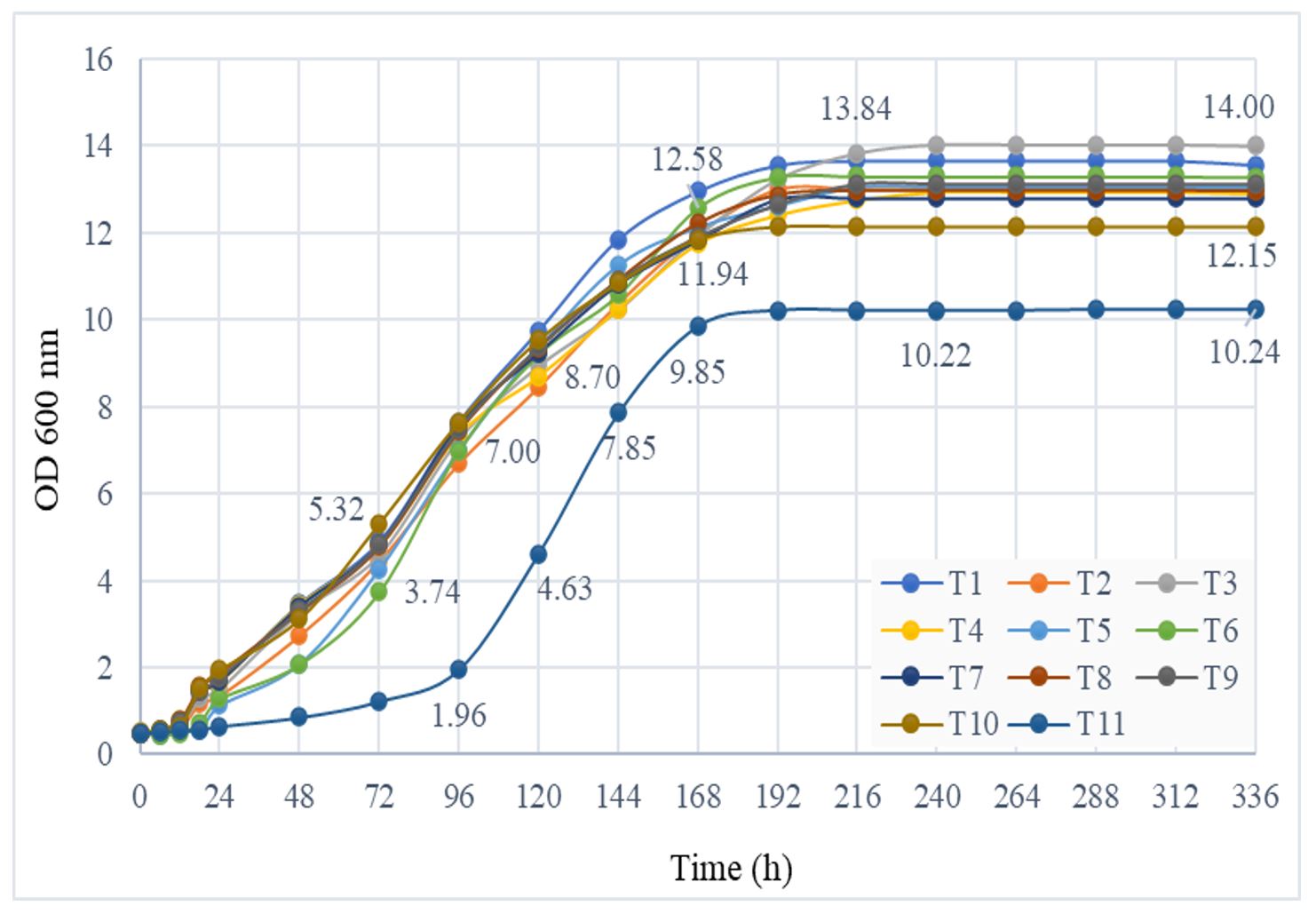
Figure 2 – Optical density at 600 nm of the yeast cultures during semi-anaerobic fermentation
Note: T1–T11 represent yeast codes as shown in Table 1.
Regarding the cell biomass at the end of the fermentation processes, it was observed that semi-anaerobiosis induced the formation of larger amounts of biomass for all strains: in anaerobiosis the biomass yield varied between 1.10 and 1.78 g/100 mL, while in semi-anaerobiosis cell biomass increased by between 5.41% (T3) and 38.20% (T4) (Figure 3).
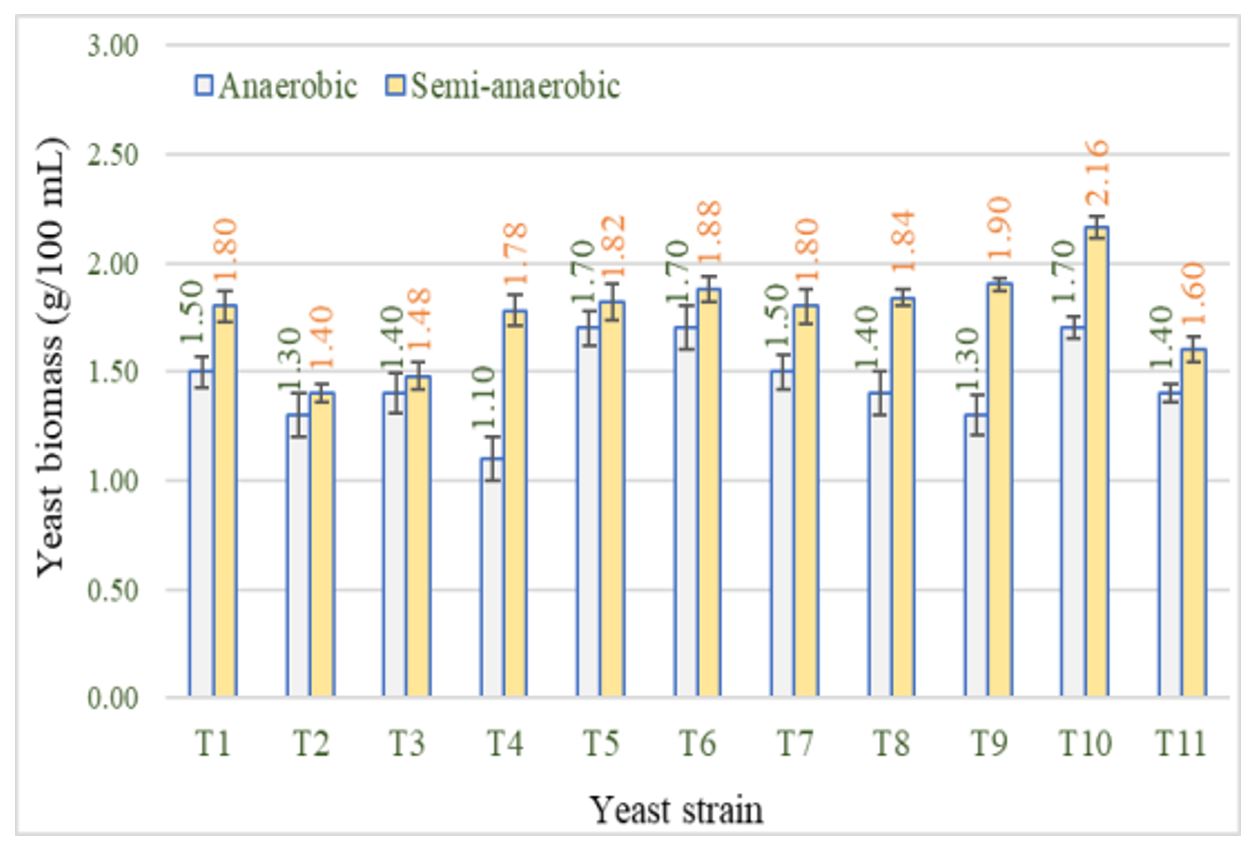
Figure 3 – Cell biomass formed by the tested yeasts in anaerobic and semi-anaerobic conditions
Note: T1–T11 represent yeast codes as shown in Table 1; error bars indicate the standard deviations (n = 2).
T. delbrueckii produced average amounts of cell biomass (1.40–1.60 g/100 mL), showing a good response to semi-anaerobiosis. Ramirez and Velázquez (2018) concluded that T. delbrueckii has less fermentation vigour and a slower growth rate than S. cerevisiae. Also, CO2 production and O2 consumption were higher in T. delbrueckii, a fact that generated lower amounts of biomass.
The alcohol yield was calculated according to the amount of sugar metabolized. Thus, in the anaerobiosis the mean values varied between 16.41 (T6) and 16.89 g (T11), while in semi-anaerobic conditions, the tested yeasts consumed between 17.44 (T4) and 19.90 g (T11) of sugar to produce 1% vol. of alcohol (Figure 4).
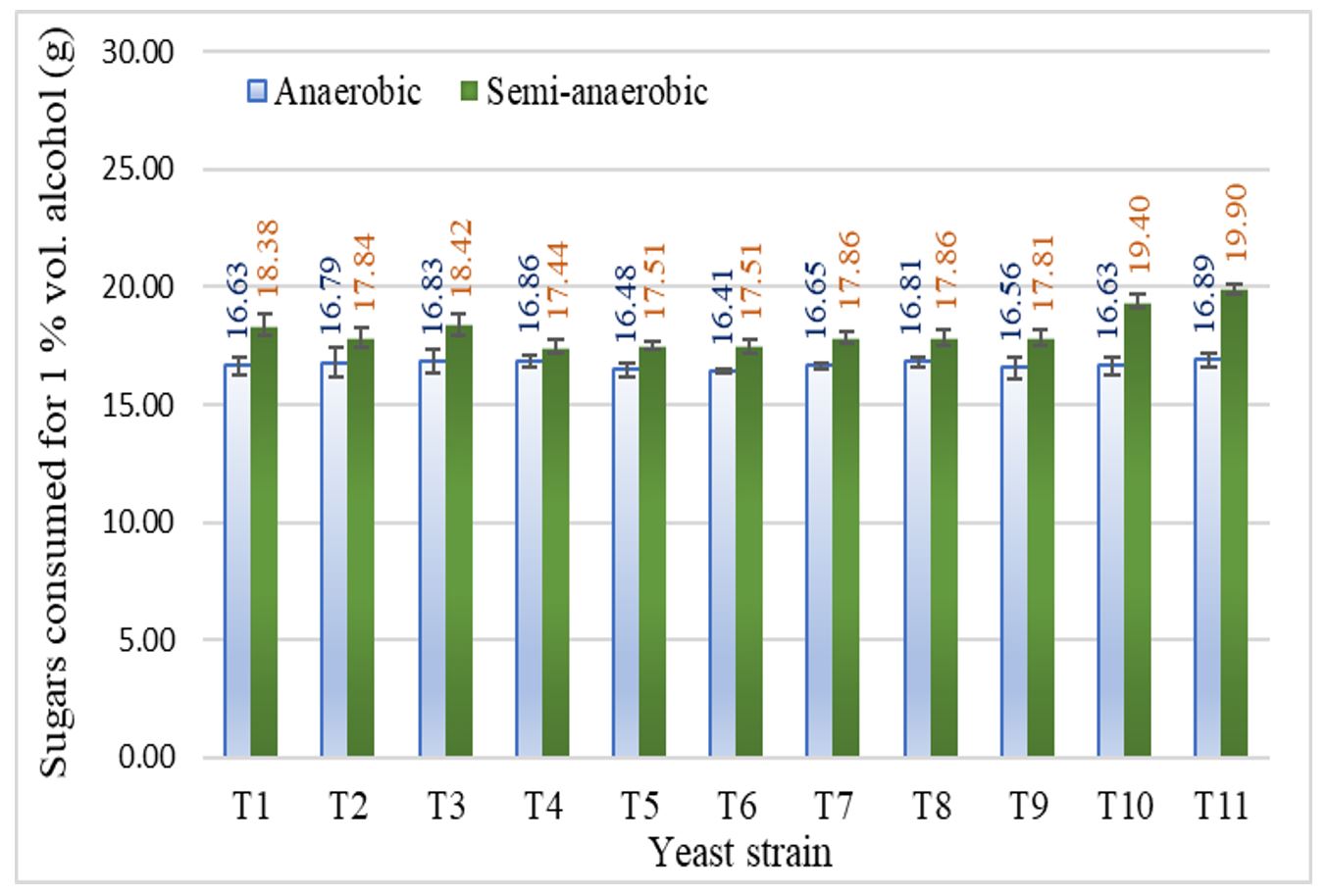
Figure 4 – Amount of sugar consumed to produce a volume of alcohol by tested yeasts
Note: T1–T11 represent yeast codes as shown in Table 1; error bars indicate the standard deviation (n = 2).
Compared to anaerobiosis, in semi-anaerobic fermentation, the Saccharomyces strains additionally consumed between 3.32% (T4) and 14.25% (T10) more sugars to produce a volume of alcohol, while the non-Saccharomyces strain (T11) consumed over 15.00% more sugar for each volume of alcohol produced.
Fermentation dominated by T. delbrueckii took longer to complete, a situation that can lead to additional wine oxidation (Ramirez and Velázquez, 2018). Values of the browning index of the wines obtained by anaerobic and semi-anaerobic fermentations, evaluated as the absorbance at 420 nm, are shown in Figure 5.
Although the recorded values were not high, a significant increase in the degree of oxidation was observed through semi-anaerobic fermentation (+35.38–54.87%), in comparison to anaerobiosis, due to the intermittent homogenization and limited gas exchange.
The complex laboratory experiments allowed the selection of two yeast strains which developed well in semi-anaerobic conditions, showed good fermentation power and consumed a larger amount of sugar for a volume of alcohol. Thus, in the micropilot experiment, the Muscat Ottonel grape must was sequentially fermented under semi-anaerobic conditions, by initial inoculation with the T. delbrueckii strain (T11) and the subsequent addition of the S. cerevisiae strain T10 (Vinoferm Aroma, Essedielle), when 50% of the sugars were consumed, in order to ensure completion of the sugar fermentation, as previously shown by Contreras et al. (2015). The control sample was anaerobically fermented in monoculture with the S. cerevisiae strain. Analysing the dynamics of yeast development (OD 600 nm), a shorter lag phase of the non-Saccharomyces strain was noticed, due to the pre-adaptation phase. After 6 days, strain T11 managed to consume 50% of the sugars, the must being inoculated with S. cerevisiae (T10), cell density increasing accordingly (Figure 6).
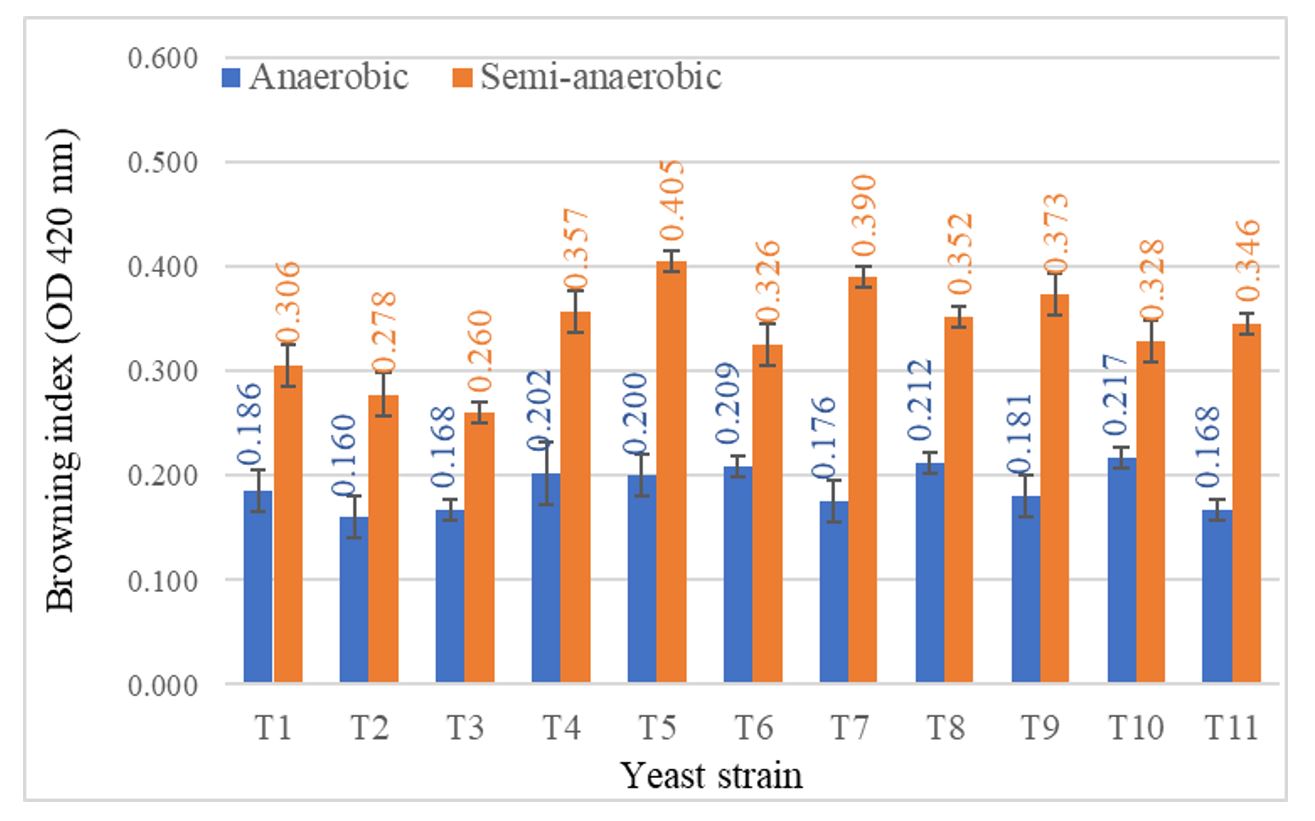
Figure 5 – Browning index of wines obtained by anaerobic and semi-anaerobic fermentations
Note: T1–T11 represent yeast codes as shown in Table 1; error bars indicate the standard deviation (n = 2).
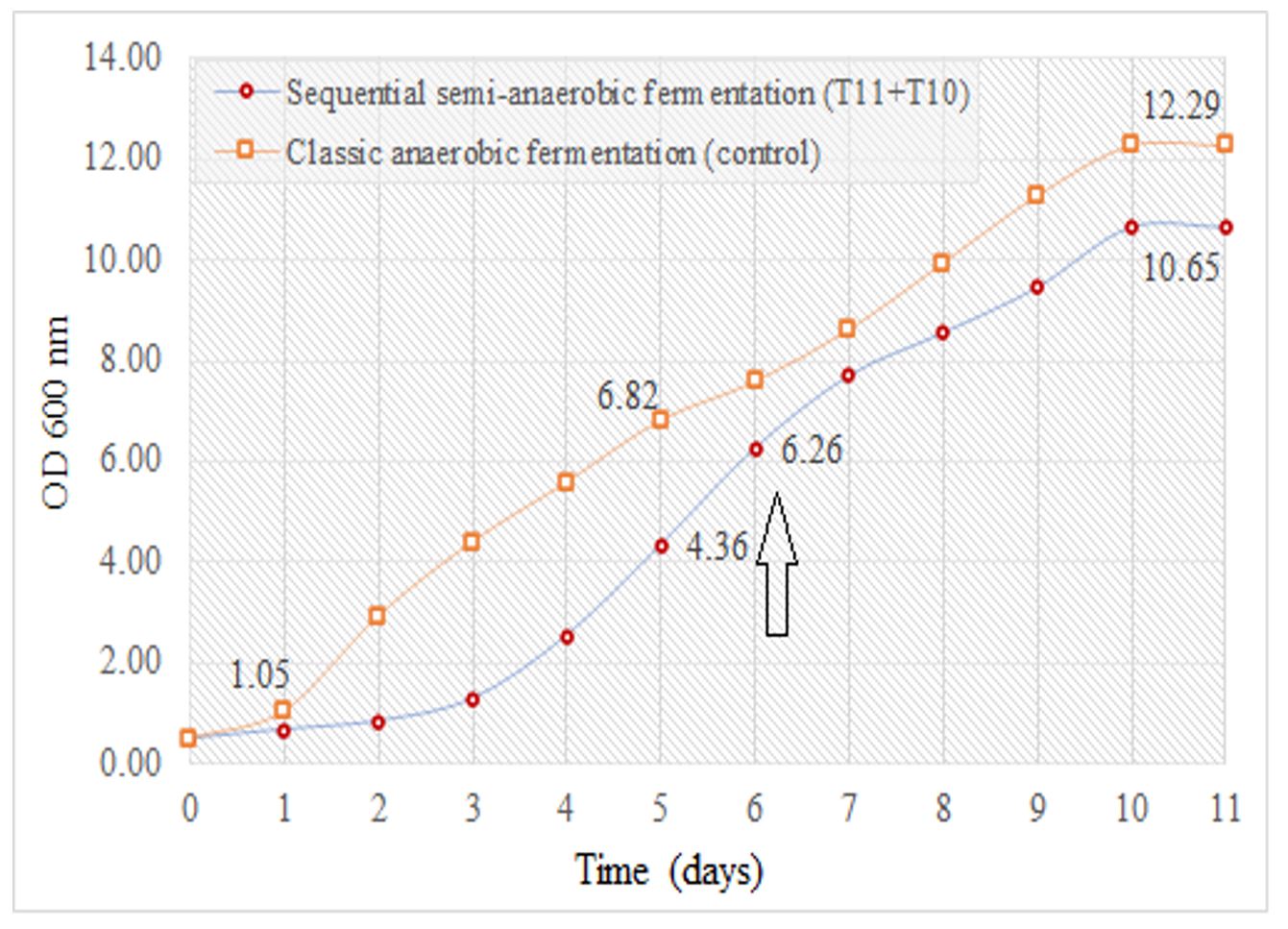
Figure 6 – Dynamics of yeast development (OD 600 nm) in sequential semi-anaerobic fermentation
Note: T10 and T11 represent yeast codes as shown in Table 1; the arrow indicates the time of S. cerevisiae strain inoculation in sequential fermentation.
After 11 days of sequential semi-anaerobic fermentation, the optical density at 600 nm was 15% lower than that of the control fermentation, probably due to microorganisms’ interactions. As known, grape must and wine constitute very complex microbial ecosystems containing a mixture of different species and strains that interact intensively during must fermentation (competition, commensalism, antagonism, parasitism) (Liu et al., 2017).
The dynamics of alcohol accumulation was different in the case of sequential semi-anaerobic fermentation, due to the distinct characteristics of the process and species involved. With the addition of S. cerevisiae, rapid completion of the alcoholic fermentation occurred (Figure 7).
The alcoholic concentration of the wines obtained by sequential fermentation in semi-anaerobiosis was lower by up to 1.10% vol. compared to classic anaerobic fermentation, meaning a decrease of about 10%, at similar residual sugar levels (dry wines).
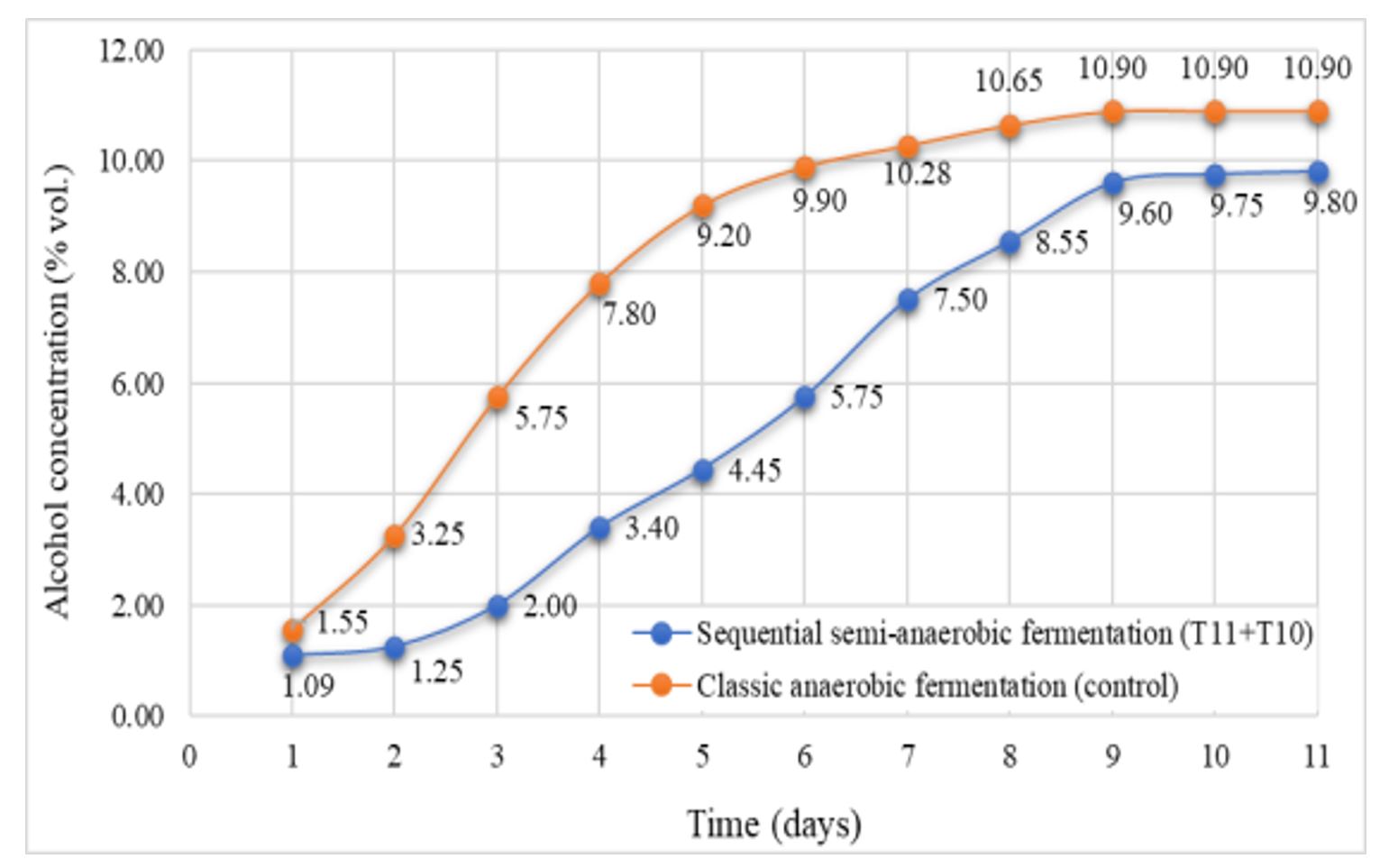
Figure 7 – Dynamics of alcohol synthesis in sequential semi-anaerobic fermentation
Note: T10 and T11 represent yeast codes as shown in Table 1.
The sugar/ethanol conversion rate varied from 16.85 (anaerobic) to 18.80 g sugar/vol. alcohol (semi-anaerobic sequential fermentation). The total acidity of the sequentially fermented wines was higher compared than that of the control wines (+0.31 g/L), while the pH was slightly lower (Table 2). The volatile acidity values of wines were very similar (0.42–0.45 g/L as acetic acid), non-significantly higher values being found in sequentially semi-anaerobic fermented wine samples. Similar results were reported by Ramirez and Velázquez (2018). The TPC of wines was low, confirmed by the absorbance at 280 nm (5.88–6.19), non-significantly lower values being recorded in wines with a lower alcohol content (Table 3). Terpenes, as aroma compounds, are present in grapes, must and wines both as free (FVT) and combined volatile compounds, especially as glycosidic aroma precursors (PVT) (Lengyel, 2012). Due to semi-anaerobic conditions, a significant reduction in FVT (−10%) was registered (Table 3).
Table 2
Physico-chemical characterization of wines obtained by semi-anaerobic sequential fermentation
Experimental variant |
Alcohol (% vol.) |
Total acidity (g/L tartaric acid) |
Volatile acidity (g/L acetic acid) |
Free SO2 (mg/L) | Total SO2 (mg/L) |
Residual sugars (g/L) |
pH |
Sequential fermentation |
9.80 ± 0.05 |
4.96 ± 0.10 |
0.45 ± 0.02 |
22 ± 1 |
107 ± 2 |
1.04 ± 0.08 |
3.11 ± 0.02 |
Control |
10.90 ± 0.05 |
4.59 ± 0.11 |
0.43 ± 0.02 |
30 ± 2 |
110 ± 4 |
0.94 ± 0.10 |
3.16 ± 0.02 |
Significance | ● | n.s. | n.s. | ● | n.s. | n.s. | n.s. |
Note: n.s. and ● – non-significant and significant at p < 0.05 (ANOVA test).
Table 3
Phenolic compounds, terpenes and glycerol in wines obtained by semi-anaerobic sequential fermentation
Experimental variant |
TPC (g GAE/L) |
TPI (OD 280 nm) |
FVT (µg LE/L) |
PVT (µg LE/L) |
Glycerol (g/L) |
Sequential fermentation | 0.26 ± 0.09 | 4.84 ± 0.08 | 298.18 ± 2.40 | 669.09 ± 6.32 | 9.32 ± 0.12 |
Control | 0.30 ± 0.11 | 5.47 ± 0.14 | 330.91 ± 6.16 | 744.24 ± 10.42 | 5.97 ± 0.17 |
Significance | n.s | ● | ● | ● | ● |
Note: TPC (GAE) – total phenolic compounds (gallic acid equivalent); FVT – free volatile terpenes (linalool equivalent); PVT – precursor volatile terpenes; TPI – total polyphenolic index (OD at 280 nm); n.s and ● – non-significant and significant at p < 0.05 (ANOVA test).
Previous studies showed that T. delbrueckii generates wines with high concentrations of terpenols, compared with wines fermented with S. cerevisiae (Sadoudi et al., 2012).
Glycerol is a component of the wine matrix closely related to yeast metabolism and contributing to the sweetness and viscosity of the wine, with a positive impact on its sensory characteristics (Nieuwoudt et al., 2002).
Semi-anaerobiosis enhanced yeast glycerol synthesis by up to 35% (Table 3). Previous studies also showed that under limited aerobic conditions the use of T. delbrueckii favours glycerol production (Contreras et al., 2015).
In the choice and consumption of food, colour plays an important role, being associated with food quality and safety. Chromatic parameters can be used in the objective description of wine
The wine obtained by semi-anaerobic fermentation showed a non-significantly higher proportion of red hue, probably due to a slight oxidation of the phenolic compounds, while the control wine showed a higher colour intensity, and higher yellow (%Y) nuances (Table 4).
For the sensory analysis of the experimental wines, 19 characteristics were assessed by the panel of wine tasters (Figure 8).
Table 4
Chromatic parameters of wines obtained by semi-anaerobic sequential fermentation
Experimental variant | Colour intensity (I) | Colour hue (H) | d 420 % (%Y) | d 520 % (%R) | d 620 % (%B) |
Sequential fermentation | 7.9 ± 0.32 | 6.2 ± 0.30 | 79.28 ± 0.29 | 15.25 ± 0.34 | 3.53 ± 0.30 |
Control | 8.0 ± 0.21 | 5.4 ± 0.24 | 81.02 ± 0.22 | 14.98 ± 0.28 | 4.00 ± 0.19 |
Significance | n.s. | n.s. | n.s. | n.s. | n.s. |
Note: %Y, %R, %B – percentage of yellow, red and blue colour; n.s. and ● – non-significant and significant at p < 0.05 (ANOVA test).
Low-alcoholic wines obtained by semi-anaerobic sequential fermentation of Muscat Ottonel must were appreciated as more acidic, with good aromas of muscat and dried fruits, and with a taste persistence and full body perception similar to those of control wine. The early oxidation of must was associated with the disappearance of green colour shades, resulting in yellow-gold tones, the lessening of some primary aromas, appearing aromas of ripe fruit and a sensation of dryness.
CONCLUSIONS
Microbiological strategies for the production of low-alcohol wines may represent a promising alternative to the currently used post-fermentation processes. By applying sequential fermentation, initiated with a T. delbrueckii yeast strain and completed with selected S. cerevisiae strains, in semi-anaerobic conditions, dry wines were obtained with an alcohol content lower by 1.10% vol., with a lower concentration of volatile terpenes and a higher amount of glycerol (< 35%). Nevertheless, sequential semi-anaerobic fermentation can be recommended for a reduction in ethanol content of 1% to 3% vol., based on the ability of non-Saccharomyces strains to perform in partial aeration conditions, maintaining an analytical and sensorial profile very similar to that of wine obtained by conventional winemaking technology. Further studies are necessary in order to select or to isolate new yeast strains capable of consuming more sugar per volume of produced alcohol, and better adapted to the semi-anaerobic or even aerobic conditions.
Author Contributions: Conceptualization: VRF and VVC; methodology: VRF, RMF and FDR; analysis VRFand AN; investigation: LR and AN; writing: VRF and RMF; review: VVC and LR. All authors declare that they have read and approved the publication of the manuscript in this present form.
Funding: This paper was published under the frame of ADER 7.5.5/30.09.2019 project, financed by the Ministry of Agriculture and Rural Development of Romania.
Conflicts of Interest: All authors declared no conflict of interest.
REFERENCES
Aceituno, F.F.; Orellana, M.; Torres, J.; Mendoza, S.; Slater, A.W.; Melo, F.; Agosin, E. Oxygen response of the wine yeast Saccharomyces cerevisiae EC1118 grown under carbon-sufficient, nitrogen-limited enological conditions. Applied and Environmental Microbiology. 2012, 78, 8340-8352. https://doi.org/10.1128%2FAEM.02305-12.
Alves-Araujo, C.; Pacheco, A.; Almeida, M.J.; Spencer-Martins, I.; Leao, C.; Sousa, M.J. Sugar utilization patterns and respiro-fermentative metabolism in the baker’s yeast Torulaspora delbrueckii. Microbiology. 2007, 153, 898-904. https://doi.org/10.1099/mic.0.2006/003475-0.
Canonico, L.; Solomon, M.; Comitini, F.; Ciani, M.; Varela, C. Volatile profile of reduced alcohol wines fermented with selected non-Saccharomyces yeasts under different aeration conditions. Food Microbiology. 2019, 84, 103247, https://doi.org/10.1016/j.fm.2019.103247.
Castelli, T. Yeasts of wine fermentations from various regions of Italy. American Journal of Enology and Viticulture. 1955, 6, 18-20.
Contreras, A.; Hidalgo, C.; Schmidt, S.; Henschke, P.A.; Curtin, C.; Varela, C. The application of non-Saccharomyces yeast in fermentations with limited aeration as a strategy for the production of wine with reduced alcohol content. International Journal of Food Microbiology. 2015, 205, 7-15. https://doi.org/10.1016/j.ijfoodmicro.2015.03.027.
Dimitriadis, E.; Williams, P.J. The development and use of a rapid analytical techniques for estimation of free potentially volatile monoterpene flavoring of grapes. American Journal of Enology and Viticulture. 1984, 35, 68-71. https://doi.org/10.5344/ajev.1984.35.2.66.
Filimon, V.R.; Sandu-Ville, Ș.; Bora, F.D.; Tudor, G.; Filimon, R.; Nechita, A.; Damian, D. Methods for producing low-alcohol wine I. Viticultural and pre-fermentation strategies. Scientific Papers USAMV Iași – Horticulture Series. 2020, 63, 95-100.
Fleet, G.H.; Heard, G.M. Yeast-growth during fermentation. In: Fleet G.H., (ed.), Wine microbiology and biotechnology. Harwood Academic, Lausanne, 1993, 27-54.
García, M.; Esteve-Zarzoso, B.; Cabellos, J.M.; Arroyo, T. Sequential non-Saccharomyces and Saccharomyces cerevisiae fermentations to reduce the alcohol content in wine. Fermentation. 2020, 6, 60. https://doi.org/10.3390/fermentation6020060.
Glories, Y. The color of red wines. 2nd part. Measurement, origin and interpretation (in French). OENO One. 1984, 18, 253-271. https://doi.org/10.20870/oeno-one.1984.18.4.1744.
Gonzalez, R.; Quirós, M.; Morales, P. Yeast respiration of sugars by non-Saccharomyces yeast species: A promising and barely explored approach to lowering alcohol content of wines. Trends in Food Science & Technology. 2013, 29, 55-61. https://doi.org/10.1016/j.tifs.2012.06.015.
Lengyel, E. Primary aromatic character of wines. Acta Universitatis Cibiniensis, Series E: Food Technology. 2012, 16, 3-18.
Liu, Y.; Rousseaux, S.; Tourdot-Maréchal, R.; Sadoudi, M.; Gougeon, R.; Schmitt-Kopplin, P.; Alexandre, H. Wine microbiome: A dynamic world of microbial interactions. Critical Reviews in Food Science and Nutrition. 2017, 57, 856-873. https://doi.org/10.1080/10408398.2014.983591.
Mehlomakulu, N.N.; Hoff, J.W.; Erten, H.; Beukes, L.; Jolly, N.P. Screening non-Saccharomyces yeasts as low ethanol producing starter cultures. South African Journal of Enology and Viticulture. 2021, 42, 56-66. http://dx.doi.org/10.21548/42-1-4335.
Meiser, J.; Vazquez, A. Oxygen dependent mitochondrial formate production and the reverse Pasteur effect. BioRxiv. 2020. https://doi.org/10.1101/2020.04.10.035675.
Nadai, C.; Vendramini, C.; Carlot, M.; Andrighetto, C.; Giacomini, A.; Corich, V. Dynamics of Saccharomyces cerevisiae strains isolated from vine bark in vineyard: influence of plant age and strain presence during grape must spontaneous fermentations. Fermentation. 2019, 5, 62. https://doi.org/10.3390/fermentation5030062.
Nieuwoudt, H.H.; Prior, B.A.; Pretorius, S.; Bauer, F.F. Glycerol in South African table wines: an assessment of its relationship to wine quality. South African Journal of Enology & Viticulture. 2002, 23, 22-30. https://doi.org/10.21548/23-1-2151.
OIV. Resolution OIV-OENO 370-2012. Guidelines for the characterization of wine yeasts of the genus Saccharomyces isolated from vitivinicultural environments. International Organization of Vine and Wine (OIV), Paris. 2012.
OIV. Compendium of international methods of wine and must analysis. Vol. 1. International Organization of Vine and Wine (OIV), Paris. 2019.
Ramirez, M.; Velázquez, R. The yeast Torulaspora delbrueckii: an interesting but difficult-to-use tool for winemaking. Fermentation. 2018, 4, 94. https://doi.org/10.3390/fermentation4040094.
Romano, P.; Braschi, G.; Siesto, G.; Patrignani, F.; Lanciotti, R. Role of yeasts on the sensory component of wines. Foods. 2022, 11, 1921. https://doi.org/10.3390/foods11131921.
Sadoudi, M.; Tourdot-Marechal, R.; Rousseaux, S.; Steyer, D.; Gallardo-Chacón, J.; Ballester, J.; Vichie, S.; Guérin-Schneider, R.; Caixach, J.; Alexandre, H. Yeast-yeast interactions revealed aromatic profile analysis of Sauvignon Blanc wine fermented by single or co-culture of non-Saccharomyces and Saccharomyces yeasts. Food Microbiology. 2012, 32, 243-253. https://doi.org/10.1016/j.fm.2012.06.006.
Academic Editor: Dr. Mihaela Roșca
Publisher Note: Regarding jurisdictional assertions in published maps and institutional affiliations ALSE maintain neutrality.
Bora Florin Dumitru, Cotea Valeriu V., Filimon Roxana Mihaela, Filimon Vasile Răzvan, Nechita Ancuța, Rotaru Liliana